Jump to a key chapter
Understanding Axon Elongation
Axon elongation is a crucial process in neural development, where nerve cells, or neurons, extend their axons to connect with other neurons, forming complex neural networks. It's essential to understand this process as it plays a significant role in brain function and repair.
The Role of Axon Elongation in the Nervous System
Axon elongation is fundamental in the development and maintenance of the nervous system. Its primary function is to enable the transmission of electrical impulses across long distances within the body.
- Communication: Axons transmit signals between neurons, vital for sensation, thought, and movement.
- Plasticity: They help adapt brain functions to new experiences and learning.
- Repair: In case of injury, axon elongation can facilitate recovery by forming new connections.
Axon Elongation: This is the process in which a neuron's axon grows longer, creating the necessary connections for efficient communication within the nervous system.
Mechanisms of Axon Elongation
Axon elongation involves several mechanisms, driven by both intrinsic cellular signals and external cues that guide the axon's growth direction. These can include:
- Growth Cone Dynamics: The growth cone at the axon's tip senses the environment and directs axon pathfinding.
- Cytoskeletal Changes: Microtubules and actin filaments remodel to push the growth cone forward.
- Signaling Pathways: Molecular signals like neurotrophins activate pathways that encourage elongation.
When studying damaged nerve regeneration, researchers often explore how axon elongation can be stimulated to restore lost connections and regain function. This demonstrates the potential applications of understanding this biological process.
Factors Influencing Axon Elongation
Several factors influence axon elongation, affecting its efficiency and success:
- Genetic Factors: Genes determine the production of proteins and receptors guiding elongation.
- Environmental Signals: Proteins in the extracellular matrix can promote or inhibit growth.
- Injury and Repair: After an injury, the nervous system can attempt to restore connections through axon growth, influenced by cytokines and other repair signals.
- Age: Younger organisms typically have a higher potential for axon elongation compared to older ones due to more active growth processes.
Interestingly, certain therapies aim to mimic the young nervous system environment to enhance axon elongation even in adults.
Challenges and Future Directions
Despite advancements in understanding axon elongation, challenges remain in translating this knowledge into effective therapeutic strategies. The complexity of the neural environment can hinder axon growth, making it difficult to replicate the process externally. Future research focuses on:
- Developing biomimetic scaffolds that support axon elongation.
- Applying gene therapy to correct or enhance growth signals.
- Exploring stem cell therapy for repairing damaged nerves.
A fascinating area of study is the role of long non-coding RNAs (lncRNAs) in axon guidance and elongation. These RNA molecules do not encode proteins but regulate gene expression at multiple levels. Recent research suggests they may play vital roles in modulating the growth cone responses to guidance cues, offering new insights into neurodevelopmental processes. Understanding this can open up innovative therapeutic pathways for nervous system repair and regeneration, highlighting the intricate dance between genetic regulation and physical axon growth.
Axon Elongation Mechanisms
Axon elongation is a fundamental biological process that ensures proper neural connectivity by extending the axons of neurons. This activity is crucial in developing a functional nervous system and maintaining neural network integrity.
Key Biological Processes
Understanding the biological processes involved in axon elongation is essential. Here are some of the main components involved in this mechanism:
- Growth Cone Dynamics: The growth cone is the motile structure at the axon's tip, actively seeking guidance cues to direct axon pathfinding. It plays a pivotal role in determining the direction and rate of axon elongation.
- Cytoskeletal Rearrangement: Actin filaments and microtubules in the cytoskeleton constantly reorganize, pushing the growth cone forward to facilitate new axon growth. This dynamic structure supports and guides elongation.
- Guidance Cues: Extracellular signals, such as chemical cues, provide directional information for growth, helping axons find their targets.
- Intracellular Signaling: Signaling pathways, often initiated by external cues, activate intracellular processes that drive cytoplasmic extensions.
Growth Cone: A dynamic, specialized region at the tip of a growing axon that responds to environmental cues and aids in directional movement.
Influential Factors and Conditions
Several factors determine the success of axon elongation. These include:
- Genetic Predispositions: Certain genes encode proteins that are essential for axon guidance and elongation.
- Extracellular Environment: The surrounding environment, composed of extracellular matrix proteins, can either promote or inhibit elongation.
- Age and Development Stage: Younger organisms show greater potential for axon elongation due to more active cellular mechanisms.
- Injury Response: After neural injuries, specific factors are released to stimulate axon regeneration.
Some environmental factors, such as neurotrophins, can accelerate axon elongation by providing positive growth signals.
Modern Research and Tools
Advancements in research tools have significantly enhanced the understanding of axon elongation mechanisms.
- Live Imaging Technology: Provides real-time visualization of axon growth, helping researchers understand growth cone behavior.
- Genetic Engineering: Techniques like CRISPR enable the manipulation of genes involved in axon elongation, allowing for detailed studies.
- Biomimetic Materials: These materials, designed to mimic the physical properties of neural environments, are being explored to support axon elongation in vitro.
Recent studies have highlighted the role of microRNA (miRNA) in axon elongation. These small non-coding RNAs are known to regulate gene expression post-transcriptionally, which can significantly impact neural development. Specific miRNAs can modulate pathways involved in cytoskeletal dynamics, thus influencing axon growth. This discovery opens up exciting possibilities for therapeutic interventions targeting miRNA pathways to promote axon regeneration in injuries.
Factors Affecting Axon Elongation
Axon elongation is influenced by a variety of factors that can enhance or impede the process. Understanding these factors is pivotal as it aids in deciphering how neurons establish connections and adapt to new circumstances.Below are key considerations in environmental and molecular contributions that affect axon elongation, detailing how these elements interact within the nervous system.
Environmental and Cellular Influences
The surrounding environment and cellular architecture play a significant role in the growth and directionality of axon elongation.
- Extracellular Matrix (ECM) Components: The ECM provides structural support and releases growth cues. It includes proteins like collagens and laminins that guide or stabilize axon growth.
- Substrate Adhesion: Axons require adhesion molecules to move along surfaces. Molecules like integrins help maintain the axon’s interaction with its environment.
- Cell-to-Cell Interactions: Axons often elongate in response to signals from neighboring cells. This includes contact-mediated attraction or repulsion, navigating axon pathways.
In a lab setting, neurons tend to grow their axons toward artificially high concentrations of laminin, demonstrating the role of substrate preference in axon elongation.
Extracellular Matrix (ECM): A complex network of proteins and carbohydrates surrounding cells that provide structural and biochemical support.
Adjustments in ECM composition can significantly alter the direction and rate of axon elongation, making it a potential target for regenerative therapies.
Molecular Contributions
Several molecular elements contribute internally and externally to the regulation of axon elongation, signaling growth or suppression.
- Neurotrophins: These protein factors, such as nerve growth factor (NGF) and brain-derived neurotrophic factor (BDNF), stimulate axon growth by activating Trk receptor signaling pathways.
- Guidance Molecules: Chemical gradients from substances like netrins, slits, and ephrins direct the growth cone toward or away from specific regions.
- GTPases: Small GTP-binding proteins such as Rho, Rac, and Cdc42 regulate cytoskeleton remodeling, essential for the growth cone movement and stability.
An intriguing factor in axon elongation is the role of gradient sensing by growth cones, which involves multiple receptors and complex signaling cascades. The ability of growth cones to integrate conflicting signals allows for precise navigation through the neural landscape. Understanding these mechanisms at a deeper level is crucial for advancing therapies aimed at nerve regeneration and repair.
Cell Signaling in Axon Elongation
The intricacies of cell signaling in axon elongation are vital for understanding how neurons grow, navigate their environment, and form the necessary connections to create complex neural networks. These signaling pathways coordinate various cellular activities to ensure successful axon extension.
Key Signaling Pathways
Numerous signaling pathways are involved in the regulation of axon elongation, each contributing to specific phases of the growth process. Some of the critical pathways include:
- Neurotrophin Signaling: Neurotrophins activate receptors that initiate signaling cascades, such as the Trk receptor pathways, crucial for survival and elongation.
- MAPK/ERK Pathway: This pathway transduces extracellular signals and affects gene expression, cytoskeletal dynamics, and cell growth.
- PI3K/Akt Pathway: Plays a major role in promoting cell survival, growth, and axon elongation through various downstream effectors.
Cell Signaling: A complex system of communication that governs basic cellular activities and coordinates cell actions.
For instance, the application of brain-derived neurotrophic factor (BDNF) in neuron cultures can enhance axon outgrowth through activation of the TrkB signaling pathway, demonstrating the crucial impact of neurotrophin signaling in axon elongation.
Roles and Effects of Signaling Molecules
Signaling molecules are pivotal in cell communication, relaying messages between and within cells to drive axon elongation. Key molecules include:
- Calcium Ions (Ca2+): Intracellular Ca2+ levels modulate the activity of various kinases and phosphatases that influence the cytoskeleton and growth cone dynamics.
- Cyclic AMP (cAMP): Acts as a secondary messenger, often enhancing neurite outgrowth by influencing gene expression and cytoskeletal reorganization.
- Lipids: Lipid signaling molecules, such as sphingosine-1-phosphate, regulate actin and microtubule dynamics, impacting cell polarity and directionality of growth.
Calcium signaling is intricate, influencing not only growth but also synaptic formation and function during neural development.
Advanced Insights into Signal Integration
Understanding how neurons integrate complex cellular signals to coordinate axon direction and elongation provides insights into not just development but potential therapeutic strategies. Signaling networks often interact or 'cross-talk' to fine-tune responses. This is crucial for adapting to changing conditions and stimuli in the neural environment.
- Src-family kinases and their role in integrating signals from multiple receptor pathways.
- Interactions between RTKs (Receptor Tyrosine Kinases) and G-protein coupled receptor signaling cascades.
- The importance of feedback loops in modulating activity and ensuring precise growth responses.
A fascinating area of exploration is the role of non-coding RNAs in signal modulation during axon elongation. These RNAs don't encode proteins but instead regulate the expression of genes associated with growth and guidance. Investigations into microRNA and long non-coding RNA reveal they are integral in adapting cellular responses to external cues, highlighting yet another layer of regulation in neural development.
Defects in Axonal Elongation
Axonal elongation is essential for the development and maintenance of neural pathways. However, defects in this process can lead to severe neurological issues, affecting both structure and function of the nervous system. Understanding these defects is crucial for developing potential treatments for associated disorders.
Causes of Axon Elongation Defects
Several factors can contribute to the occurrence of defects in axonal elongation, disrupting the normal growth and connectivity of neurons.
- Genetic Mutations: Mutations in genes that encode proteins involved in axonal guidance and cytoskeletal remodeling can severely impair axon growth.
- Environmental Toxins: Exposure to toxic substances during critical periods of neural development can disrupt the molecular pathways that guide axon elongation.
- Nutritional Deficiencies: Inadequate levels of essential nutrients like folic acid or vitamin B12 can impede neuronal growth pathways.
- Metabolic Disorders: Conditions such as diabetes can alter glucose metabolism, impacting the energy supply required for axon extension.
Fragile X syndrome is a genetic disorder resulting from a mutation in the FMR1 gene, which affects synaptic plasticity and axonal growth, demonstrating the impact of genetic defects on neural development.
Research shows that some neurodegenerative diseases might trace their origins to defects in early axonal elongation processes.
Impact on Neuronal Migration
Defects in axon elongation can substantially affect neuronal migration, which is the movement of neurons to their destined positions during brain development. Such defects can lead to:
- Misrouting of Axons: Incorrect pathfinding can cause axons to establish erroneous synaptic connections.
- Delayed Migration: Neurons may take longer to reach their targets, affecting the timing and integration into neural circuits.
- Neurodevelopmental Disorders: Impaired neuronal migration is linked to conditions such as autism spectrum disorders and schizophrenia.
Recent studies investigate how defects in axonal elongation may lead to disrupted cortical lamination, a process crucial for the organized structure of the brain. This disorganization can result in functional impairments and is frequently observed in certain cognitive disorders. Understanding the tissue and molecular interactions involved could provide insights into novel therapeutic approaches for addressing these developmental challenges.
Techniques for Studying Axon Elongation
To explore the mechanisms of axon elongation, researchers employ various techniques that provide insight into the dynamics of neural growth. These methods are essential for investigating how neurons extend their axons to form functional networks in the nervous system. Below, several key techniques are outlined, showcasing how they contribute to our understanding of axon elongation.
In Vitro Methods
In vitro techniques provide a controlled environment to study axon elongation. These methods enable detailed manipulation and observation, crucial for understanding the fundamental aspects of neural growth.
- Primary Neuron Culture: Culturing neurons from brain tissue allows the study of their intrinsic growth properties.
- Microfluidic Devices: These devices can create gradients of guidance molecules to observe axon response to directional cues.
- Time-Lapse Imaging: Live-cell imaging with fluorescent markers enables visualization of axon elongation dynamics.
In an example of using primary neuron culture, researchers can add various growth factors to the culture medium to observe changes in axon growth rates and patterns.
In Vivo Techniques
Studying axon elongation in vivo offers insights into how these mechanisms operate within the complex environment of a living organism.
- Genetically Modified Models: Animals with specific gene knockouts or overexpression provide data on gene function in axonal growth.
- In Vivo Imaging: Techniques like two-photon microscopy allow visual tracking of axons as they grow in real-time within an organism.
- Electrophysiological Recording: Measures neuron activity and growth response to various stimuli.
Using techniques like in vivo imaging allows researchers to observe axonal behavior in its natural context, providing insight into cellular interactions within the brain.
Biochemical and Molecular Techniques
Biochemical approaches offer molecular insight into the cellular processes of axon elongation:
- Western Blotting: Analyzes proteins involved in axonal growth or guidance pathways.
- CRISPR Gene Editing: Modifies genes to examine their role in axon elongation.
- RNA Sequencing: Identifies gene expression patterns related to axonal growth factors.
An intriguing area of research involves the use of optogenetics to study axon elongation. This technique uses light to control cells within living tissue, typically neurons, that have been genetically modified to express light-sensitive ion channels. With this method, researchers can manipulate neuronal activity and study its direct effects on axon elongation and synaptic development, offering unparalleled precision in understanding the dynamic processes of neural networks.
axon elongation - Key takeaways
- Definition of Axon Elongation: A process where a neuron's axon grows longer to establish neural connections, vital for neural network development and repair.
- Axon Elongation Mechanisms: Involves growth cone dynamics, cytoskeletal changes, and signaling pathways (e.g., neurotrophins) to guide axon growth.
- Factors Affecting Axon Elongation: Influenced by genetic factors, environmental cues, injury response, age, and nutritional factors, which can enhance or impede growth.
- Defects in Axonal Elongation and Neuronal Migration: Can result from genetic mutations, environmental toxins, and metabolic disorders, impacting neural connectivity and disorders like autism.
- Cell Signaling in Axon Elongation: Critical pathways include the neurotrophin signaling, MAPK/ERK, and PI3K/Akt, coordinating cellular processes for axon growth.
- Axon Elongation Techniques: Include in vitro methods like neuron culture and microfluidic devices, in vivo imaging, and biochemical approaches like CRISPR and RNA sequencing.
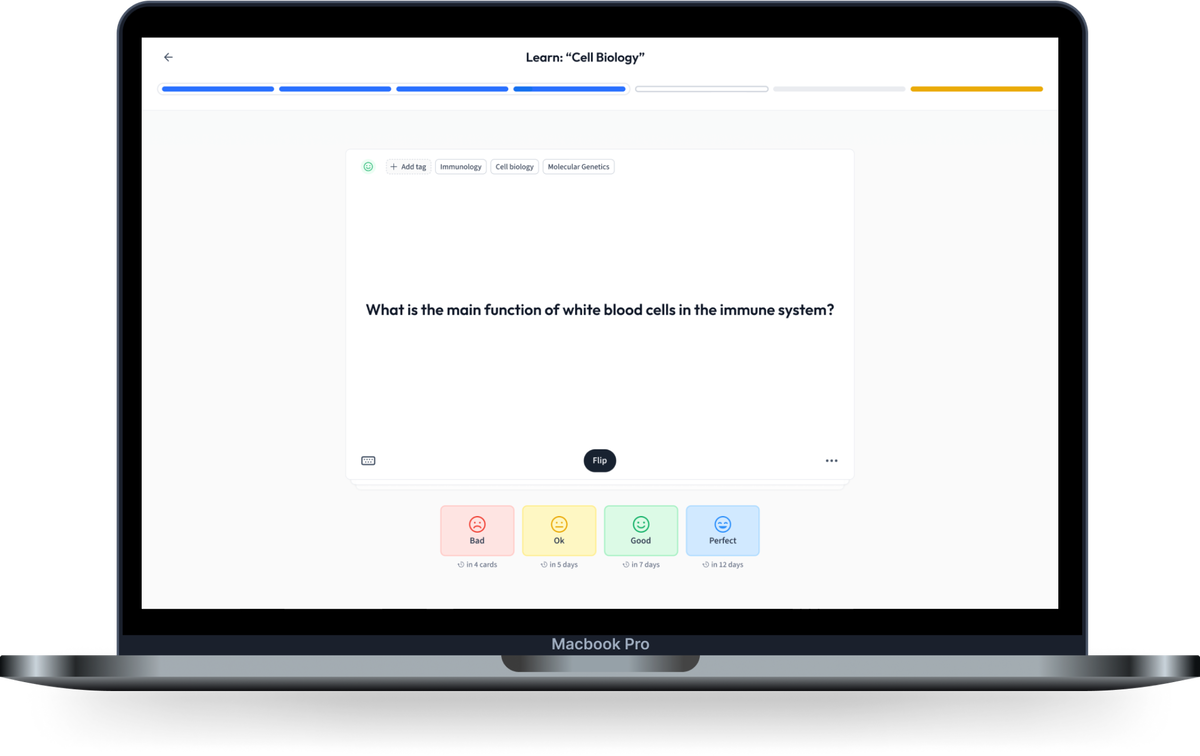
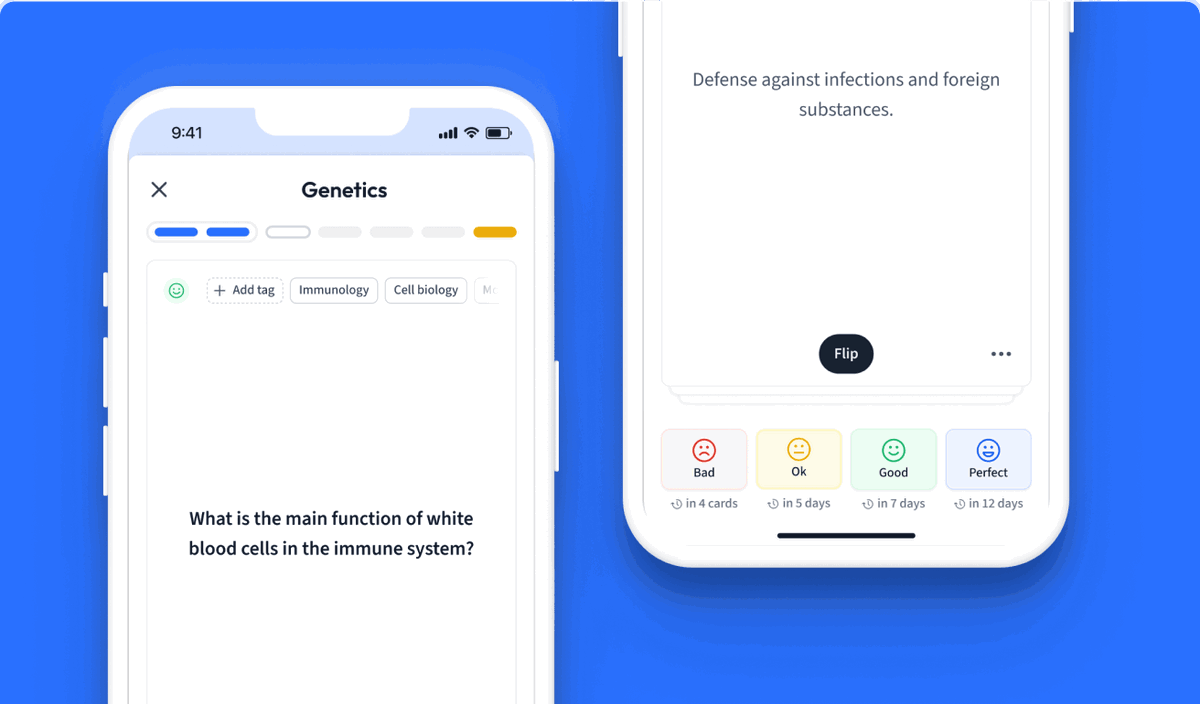
Learn with 12 axon elongation flashcards in the free StudySmarter app
Already have an account? Log in
Frequently Asked Questions about axon elongation
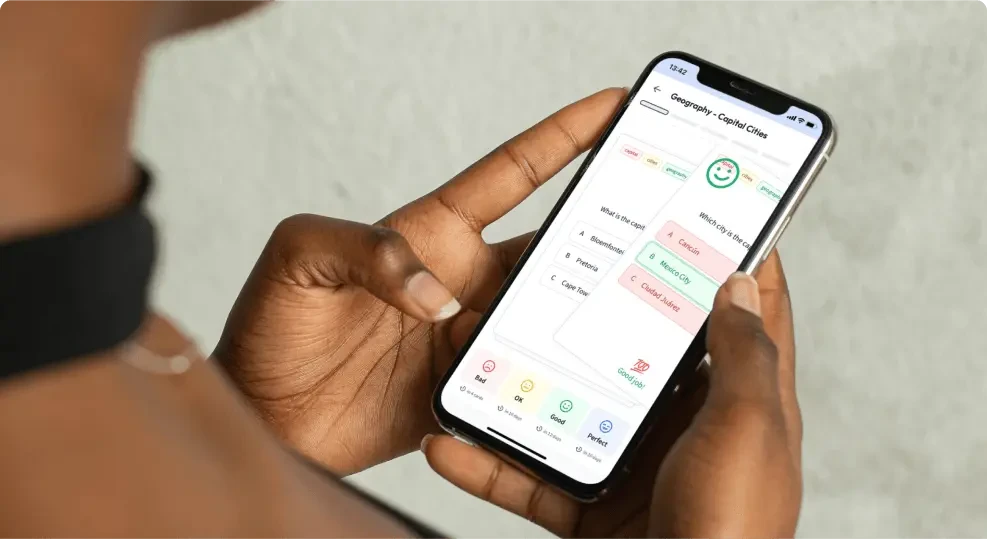
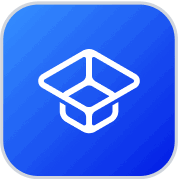
About StudySmarter
StudySmarter is a globally recognized educational technology company, offering a holistic learning platform designed for students of all ages and educational levels. Our platform provides learning support for a wide range of subjects, including STEM, Social Sciences, and Languages and also helps students to successfully master various tests and exams worldwide, such as GCSE, A Level, SAT, ACT, Abitur, and more. We offer an extensive library of learning materials, including interactive flashcards, comprehensive textbook solutions, and detailed explanations. The cutting-edge technology and tools we provide help students create their own learning materials. StudySmarter’s content is not only expert-verified but also regularly updated to ensure accuracy and relevance.
Learn more