Jump to a key chapter
Axon Potential Definition and Meaning
Understanding axon potentials is crucial in the field of neurobiology as it involves the fundamental processes that underpin nerve signal transmission within the nervous system. Axon potentials are essential for the communication between neurons, allowing for the transmission of signals from one part of the body to another.
What is an Axon Potential?
An axon potential, also known as an action potential, is a momentary reversal of the electric polarization of the membrane of a nerve cell (neuron) or muscle cell. Axon potentials enable the transfer of information in the nervous system by causing electrical impulses to travel along the nerve cells.
Axon potentials are generated when a stimulus causes the membrane potential to reach a threshold. This results in a rapid influx of sodium ions (Na+) due to the opening of sodium channels, followed by an efflux of potassium ions (K+) as potassium channels open. This process creates a quick change in voltage across the neuronal membrane, establishing an action potential.
The typical threshold potential for an axon potential to occur in neurons is around -55 mV.
The Process of Axon Potential
The generation of an axon potential involves several distinct phases:
- Resting Potential: Before an axon potential begins, the neuron is at a resting state with a resting potential of approximately -70 mV. This state is maintained by the sodium-potassium pump which actively transports Na+ out and K+ into the cell.
- Depolarization: When a stimulus reaches a neuron, it causes the sodium channels to open, allowing Na+ ions to flow into the cell. If the influx is sufficient to reach the threshold potential, depolarization occurs, further opening the sodium channels.
- Repolarization: After the peak of the action potential (\text{approximately} +30 mV), sodium channels close and potassium channels open, allowing K+ to move out of the cell, causing repolarization.
- Hyperpolarization: The efflux of K+ may temporarily exceed the resting potential, resulting in hyperpolarization before the neuron returns to its resting state.
The velocity of axon potential propagation can vary greatly, affected by factors such as axon diameter and whether the neuron is myelinated. In myelinated neurons, axon potentials propagate via saltatory conduction, where the impulse jumps from one node of Ranvier to the next, significantly increasing conduction speed compared to non-myelinated neurons.
Mathematically, the speed of conduction can be represented by the equation:
\[ v = \lambda f\]
Where:- \( v \) = conduction velocity
- \( \lambda \) = distance between nodes
- \( f \) = frequency of node-to-node impulse jumps
How Action Potentials Move Along Axons
The movement of axon potentials along axons is a vital process for the transmission of information within the nervous system. This movement occurs through a series of physiological events that ensure the rapid conveyance of nerve impulses from one end of a neuron to the other.
Movement Mechanism of Axon Potentials
The axon potential is a rapid and temporary change in the electrical potential across a neuron's membrane, allowing for the propagation of the nerve impulse along the axon. This is also known as nerve impulse propagation.
Once an axon potential is initiated at the axon hillock, it must move down the axon to the axon terminals:
- Depolarization Wave: The influx of Na+ creates a depolarization wave that travels along the axon.
- Local Currents: This wave generates local currents, depolarizing adjacent sections of the axon membrane.
- Sequential Activation: As each segment depolarizes, more Na+ channels open, continuing the wave along the axon.
- Refractory Period: Following depolarization, a refractory period prevents the backward movement of the impulse.
The speed of axon potentials can be influenced by the axon's diameter and its degree of myelination.
Role of Myelin in Axon Potential Propagation
Myelin sheaths around axons allow for saltatory conduction, where the axon potential jumps between nodes of Ranvier. This significantly increases conduction speed by minimizing axonal membrane depolarization to only those nodes:
- Denser coverage of myelin results in faster nerve impulse conduction.
- Action potentials leap over insulated myelinated segments, reducing the time for signal transmission.
- This process is essential for the rapid transmission of sensory information and motor commands.
An idealized formula to estimate conduction speed based on axonal properties might be given as:
\[v = \frac{d}{R} \cdot V_m \]
Where:- \( v \) = conduction velocity
- \( d \) = axon diameter
- \( R \) = resistance along the axon membrane
- \( V_m \) = membrane potential
Conduction of Action Potentials in Myelinated Axons
The conduction of action potentials in myelinated axons is a fascinating process that enhances the speed and efficiency of nerve signal transmission. Myelinated axons are covered with a fatty substance called myelin, which significantly influences how these nervous impulses travel.
Understanding Saltatory Conduction
Saltatory conduction is the process by which action potentials jump from one node of Ranvier to the next. This method of conduction allows for faster signal transmission compared to non-myelinated axons.
In myelinated axons, the presence of myelin sheaths prevents ion exchange over the axon's length, except at the nodes of Ranvier where the axon is exposed. This arrangement enables the following:
- Rapid Transmission: The action potential can leap from node to node, effectively bypassing the insulated sections and reducing the time and distance the signal must travel.
- Energy Efficiency: Reducing the action potential generation to only the nodes saves energy that would otherwise be spent in non-myelinated axons.
- Increased Conduction Speed: Myelin boosts the speed of conduction by allowing the electrical current to flow more efficiently.
Myelinated axons can conduct action potentials at speeds of up to 120 meters per second, compared to around 2 meters per second in non-myelinated axons.
The Role of Nodes of Ranvier
Nodes of Ranvier are crucial in the saltatory conduction process. They are regularly spaced gaps in the myelin sheath, allowing for ion exchanges across the axonal membrane needed to generate an action potential. At these nodes:
- Ion Channels Concentration: A high density of sodium and potassium channels is present, enabling rapid depolarization and repolarization.
- Electrical Reset: The electrochemical gradient is restored to prepare for the next impulse.
The hopping mechanism can be represented by the following idealized formula describing the speed of signal conduction:
\[v = \lambda \times f\]
Where:- \( v \) = conduction velocity
- \( \lambda \) = distance between nodes
- \( f \) = frequency of impulse jumps
How is an Action Potential Propagated Along an Axon
In the nervous system, the propagation of an action potential along an axon is fundamental for nerve signal transmission. This process involves a series of carefully orchestrated physiological events that ensure rapid communication between neurons.
Phases of Action Potential Propagation
The propagation occurs in stages:
- Depolarization: Initiated by a stimulus, sodium channels open, allowing Na+ ions to flow into the neuron, causing the inside to become more positive.
- Repolarization: As the peak voltage is reached, sodium channels close and potassium channels open, allowing K+ to exit the cell and restore the negative internal charge.
- Hyperpolarization: Exiting K+ causes a slight overshoot of negativity, before returning to the resting potential.
The refractory period following hyperpolarization ensures unidirectional impulse propagation.
Role of Myelin in Transmission
Myelin sheaths provide insulation around axons and enhance electrical signal transmission. This mostly happens through saltatory conduction, where the action potential jumps from one node of Ranvier to the next, enhancing speed and efficiency:
- Skipping myelinated sections significantly accelerates transmission speed.
- Conserves energy by minimizing action potential regeneration to nodes.
With Myelin | Faster conduction speed, energy efficient, node-specific depolarization |
Without Myelin | Slower conduction speed, more energy required, continuous depolarization |
In saltatory conduction, the myelin's insulating properties are pivotal. The nodes of Ranvier, gaps in the myelin, are loaded with ion channels. These allow for rapid depolarization specific to these areas:
- Each node features a high density of sodium channels, creating a quicker depolarization phase.
- The leap-frog nature of electrical transmission via the nodes results in considerable time savings in impulse conduction.
The efficiency of this process is key in higher vertebrates for rapid nervous response necessitated by complex behaviors.
axon potentials - Key takeaways
- Axon Potentials Definition: Axon potentials, also known as action potentials, are rapid changes in electrical charge across a neuron's membrane, essential for nerve signal transmission.
- Mechanism of Action Potentials: An action potential involves phases such as depolarization, repolarization, and hyperpolarization, facilitated by the movement of sodium and potassium ions across the membrane.
- Propagation Along Axon: Action potentials propagate along axons through a wave of depolarization, sequential activation of sodium channels, and the refractory period ensuring unidirectional flow.
- Conduction in Myelinated Axons: Myelinated axons use saltatory conduction, where the action potential jumps between nodes of Ranvier, significantly increasing conduction speed.
- Axon Diameter and Myelination Effects: The speed of action potential propagation is influenced by the axon's diameter and the presence of myelin sheaths.
- Myelin and Nodes of Ranvier: Myelin prevents ion exchange except at nodes of Ranvier, allowing for faster and energy-efficient signal transmission through saltatory conduction.
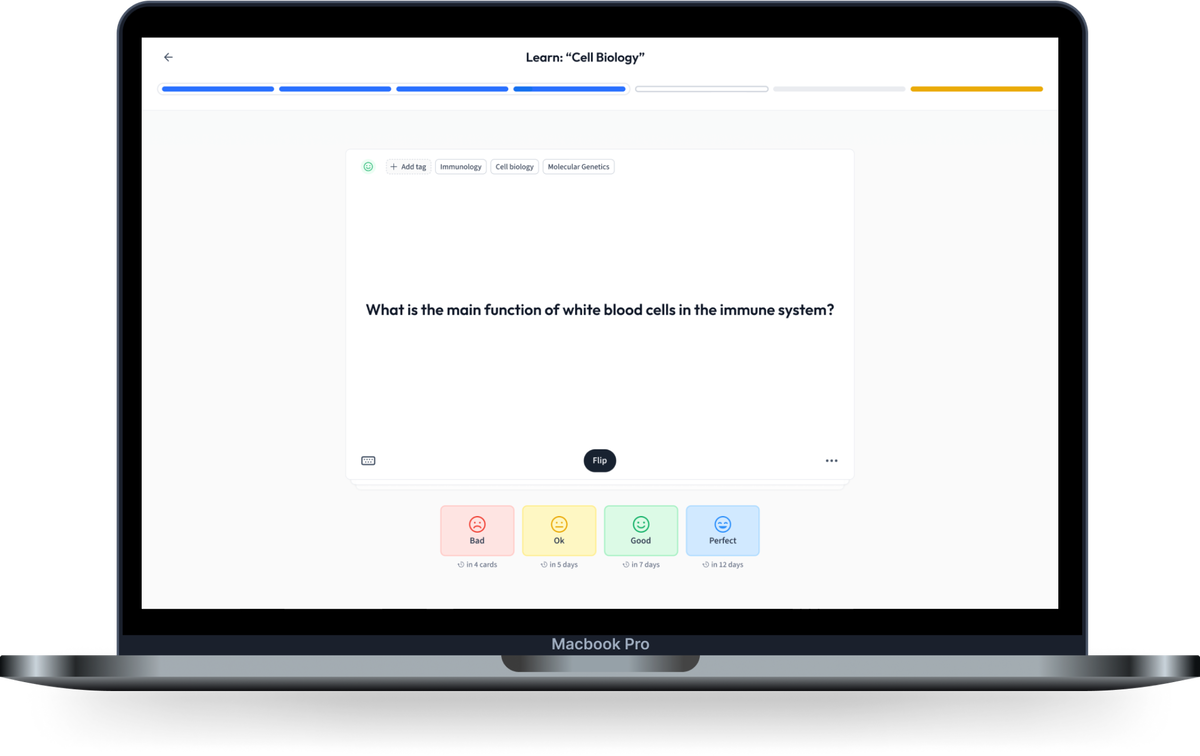
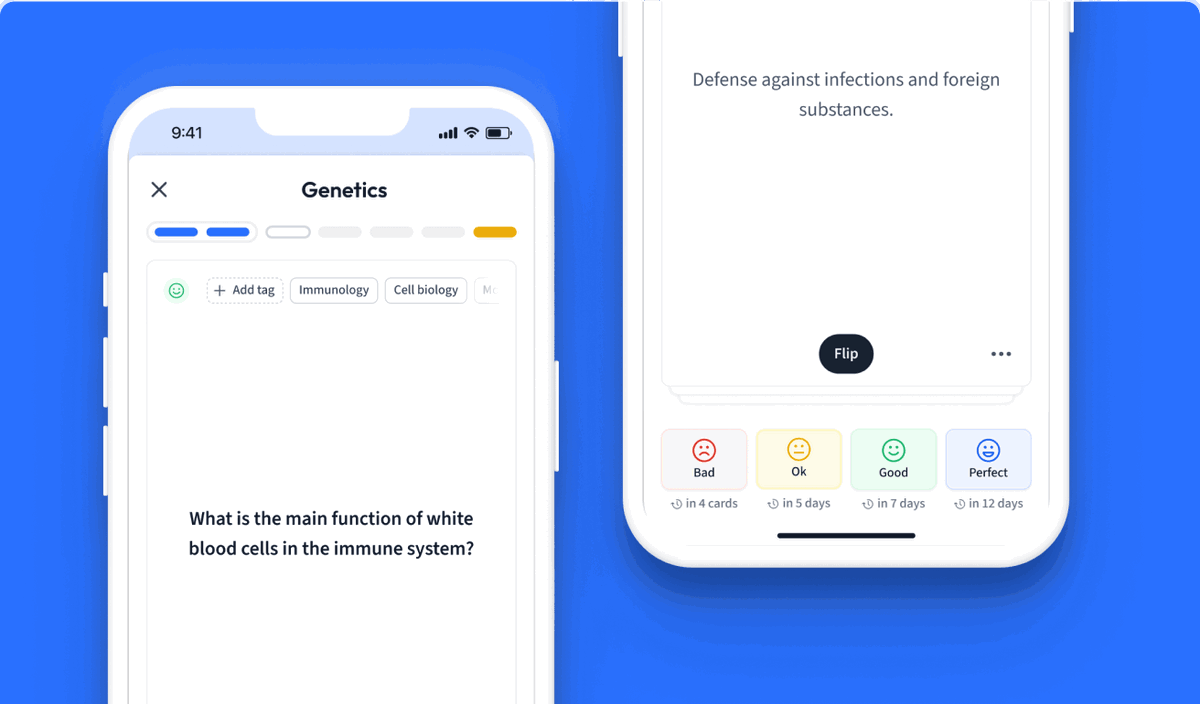
Learn with 12 axon potentials flashcards in the free StudySmarter app
Already have an account? Log in
Frequently Asked Questions about axon potentials
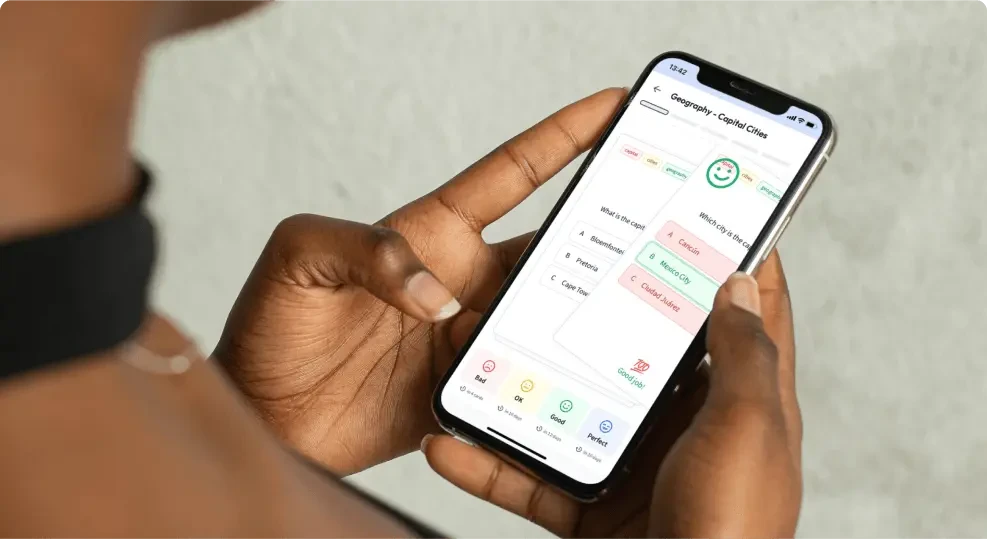
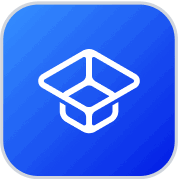
About StudySmarter
StudySmarter is a globally recognized educational technology company, offering a holistic learning platform designed for students of all ages and educational levels. Our platform provides learning support for a wide range of subjects, including STEM, Social Sciences, and Languages and also helps students to successfully master various tests and exams worldwide, such as GCSE, A Level, SAT, ACT, Abitur, and more. We offer an extensive library of learning materials, including interactive flashcards, comprehensive textbook solutions, and detailed explanations. The cutting-edge technology and tools we provide help students create their own learning materials. StudySmarter’s content is not only expert-verified but also regularly updated to ensure accuracy and relevance.
Learn more