Jump to a key chapter
Neuronal Excitability Explained
Understanding neuronal excitability is crucial for those who wish to learn about how neurons function within the nervous system. The ability of neurons to respond to stimuli and convert them into nerve impulses is what defines this excitability.
What is Neuronal Excitability?
Neuronal excitability refers to a neuron's capacity to respond adequately to stimuli and convert this stimuli into an action potential. This process is critical for communication within the nervous system.
Neurons have a resting potential, which is the electrical potential difference across their membrane when inactive. When a stimulus is sufficient to cause a depolarization that surpasses a certain threshold, an action potential is triggered, allowing the neuron to communicate with other neurons or muscles.
Consider the reflex action when you touch something hot. The sensory neurons become excited and send signals to the spinal cord, leading to an immediate reflex response to pull your hand back.
In more detailed terms, neuronal excitability involves a balance of various ions, including sodium (Na+), potassium (K+), calcium (Ca2+), and chloride (Cl−), moving across the neuronal membrane. Ion channels play a significant role in this balance, opening and closing to regulate the flow of ions, thus influencing the action potentials.
Definition of Neuronal Excitability
Neuronal excitability is a term used to define how neurons generate and transmit electrical impulses in response to stimuli. This fundamental property is what enables communication within the nervous system, playing a vital role in how organisms perceive and react to the world around them.
Neuronal excitability is the ability of a neuron to respond to a stimulus and convert it into an electrical signal, known as an action potential. This process is essential for transmitting information throughout the nervous system.
Different factors influence neuronal excitability, including the type of neuron, the state of ionic channels, and the surrounding environment. Changes in these factors can affect how easily a neuron can be excited.
For instance, during a muscle contraction, motor neurons must be excited rather quickly to transmit signals to the muscle fibers. This ensures that movements occur smoothly and efficiently.
A neuron's excitability can vary based on the presence of neurotransmitters and other chemical modulators in its vicinity.
Ion channels are pivotal in determining neuronal excitability. These proteins embedded in the neuron's membrane regulate the flow of ions such as sodium, potassium, and calcium in and out of the cell. The opening and closing of these channels during depolarization and repolarization phases are crucial for the initiation and propagation of action potentials. Hence, any malfunction in these channels can lead to various neurological disorders.
Understanding neuronal excitability helps in the study of various neurological conditions. Abnormal excitability has associations with diseases like epilepsy, where neurons become overly excitable, leading to seizures. Detecting and addressing issues with neuronal excitability can be crucial in managing such health conditions.
What Does It Mean That Neurons Are Excitable?
Neuronal excitability is an essential characteristic that allows neurons to transmit information through the nervous system. It involves the ability of neurons to generate electrical signals in response to stimuli. These signals are vital for daily functions like movement, sensation, and cognition.
Mechanisms of Neuronal Excitability
The excitability of a neuron is determined by its membrane potential and the activity of ion channels. These channels control the movement of ions like sodium (Na+) and potassium (K+) across the neuron's membrane. When a neuron receives a stimulus, it causes changes in the ion flow, leading to action potentials.
An action potential is an electrical impulse generated by a neuron in response to a stimulus. It is the primary mechanism by which neurons communicate.
Imagine you're touching something sharp. The sensory neurons in your skin become excited and send messages to your brain, which processes the sensation and prompts a quick response.
The threshold is the minimum level of stimulus required to activate a neuron and trigger an action potential.
The role of ion channels in neuronal excitability is profound. These proteins act as gatekeepers, opening and closing in response to changes in membrane potential. There are various types of ion channels, each selective for different ions. For example, sodium channels are primarily responsible for the depolarization phase of an action potential. If these channels are dysfunctional, it can lead to neurological issues like epileptic seizures. This condition occurs when neurons become overly excitable and fire excessively. Understanding the complexities of ion channels provides insight into the treatments of neurological disorders. Therapies often focus on modulating channel activity with specific drugs to manage conditions like epilepsy and chronic pain.
Mechanisms of Neuronal Excitability
At the core of neuronal excitability are the mechanisms that allow neurons to respond to stimuli. These mechanisms are primarily based on the movement of ions across the neuronal membrane through specialized ion channels. When a neuron is stimulated, ion channels open to allow the flow of ions such as sodium (Na+) and potassium (K+). This flow alters the electrical charge within the neuron, leading to the generation of an action potential.
An action potential is a rapid rise and subsequent fall in electrical potential across a neuron's membrane, allowing it to transmit an electrical signal.
The opening and closing of these ion channels depend on specific voltage changes across the neuron's membrane.
For instance, during a reflex action, such as the knee-jerk response, sensory neurons rapidly become excited, resulting in a quick and automatic muscle contraction.
The complexity of ion channels is astonishing. They have highly selective filters that ensure only specific ions pass through. These channels can be voltage-gated, ligand-gated, or mechanically gated, depending on the stimulus required to open them. Voltage-gated channels, for example, are integral to the propagation of action potentials and thus to neuronal excitability. Dysfunctions in these channels can cause various disorders, including epilepsy and cardiac arrhythmias.
Factors Influencing Excitability in Neurons
Several factors influence the excitability of neurons. Understanding these factors can help us comprehend why some neurons are more responsive to stimuli than others. Key factors include:
- Ion concentration: Changes in the concentration of ions like Na+ and K+ both inside and outside the neuron affect excitability.
- Membrane potential: The initial electrical potential of the neuron's membrane can determine its readiness to fire an action potential.
- Temperature: High or low temperatures can influence the rate of ion channel opening and closing.
- Genetic factors: Genetic mutations can alter the structure and function of ion channels, affecting excitable behavior.
Environmental factors such as toxins and drugs can also impact neuronal excitability. For instance, certain toxins from plants and animals can block ion channels, preventing action potentials. Pharmaceuticals designed to treat neurological disorders often work by modulating the activity of specific ion channels, influencing excitability in targeted neurons.
neuronal excitability - Key takeaways
- Neuronal Excitability: Refers to a neuron's capacity to respond to stimuli and convert them into action potentials, enabling communication within the nervous system.
- Action Potential: An electrical impulse generated by a neuron in response to a stimulus, allowing for communication with other neurons or muscles.
- Ion Channels: Proteins in the neuron's membrane that regulate ion flow (e.g., sodium, potassium) influencing action potentials and neuronal excitability.
- Membrane Potential: The electrical potential difference across a neuron’s membrane that plays a crucial role in excitability.
- Factors Influencing Excitability: Include ion concentration, membrane potential, temperature, and genetic factors affecting the responsiveness of neurons.
- Excitability and Disorders: Abnormal neuronal excitability is linked to conditions like epilepsy, where neurons become overly excitable.
Learn faster with the 12 flashcards about neuronal excitability
Sign up for free to gain access to all our flashcards.
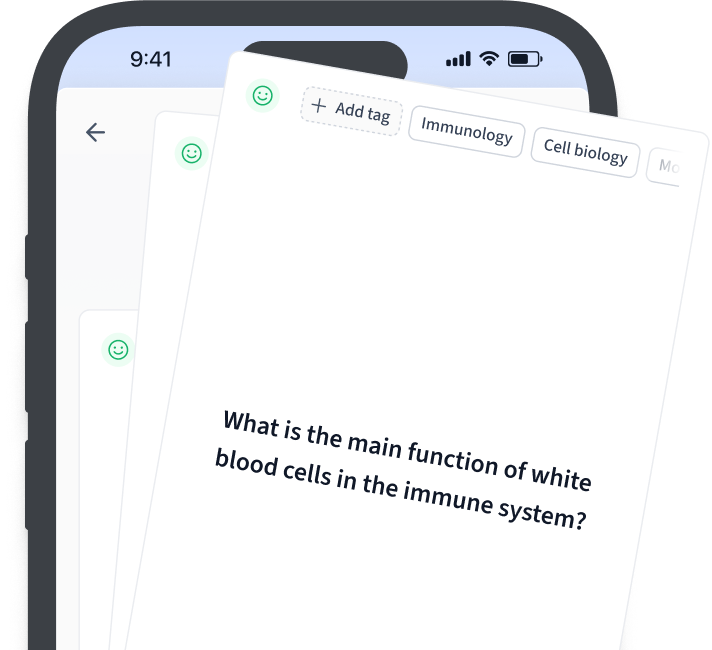
Frequently Asked Questions about neuronal excitability
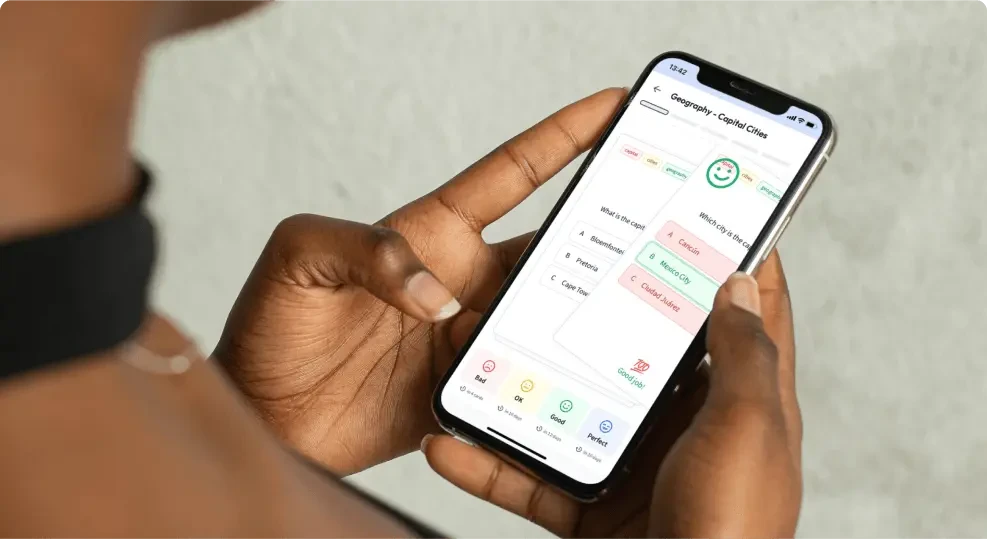
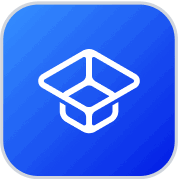
About StudySmarter
StudySmarter is a globally recognized educational technology company, offering a holistic learning platform designed for students of all ages and educational levels. Our platform provides learning support for a wide range of subjects, including STEM, Social Sciences, and Languages and also helps students to successfully master various tests and exams worldwide, such as GCSE, A Level, SAT, ACT, Abitur, and more. We offer an extensive library of learning materials, including interactive flashcards, comprehensive textbook solutions, and detailed explanations. The cutting-edge technology and tools we provide help students create their own learning materials. StudySmarter’s content is not only expert-verified but also regularly updated to ensure accuracy and relevance.
Learn more