Jump to a key chapter
Definition of Spiking Activity in Neurons
Spiking activity in neurons refers to the firing of neurons in response to stimuli. This activity is essential for the functioning of the nervous system, as it allows for communication between neurons.
Spiking activity is defined as the occurrence of electrical signals, known as action potentials, that travel along the axons of neurons. The generation of these spikes is a result of the movement of ions across the neuron's membrane.
Consider a neuron that is stimulated by a brief electrical shock. In response, it generates a sequence of spikes that can be detected using electrophysiological techniques, such as patch-clamp recordings.
The frequency and pattern of spikes are crucial in conveying different types of information between neurons.
Characteristics of Neural Spiking Activity
Neural spiking activity exhibits several interesting characteristics, which are pivotal for understanding how neurons encode and transmit information.
- Frequency: The rate at which spikes occur, measured in Hertz (Hz), determines how much information is being transmitted.
- Amplitude: Each action potential has a consistent amplitude, dictated by the properties of the ion channels involved in its generation.
- Refractory Period: After a spike, a neuron temporarily becomes less excitable during the refractory period, meaning it cannot fire another spike immediately.
- Temporal Patterns: The timing of spikes can form certain patterns that are crucial for synaptic plasticity and learning.
Understanding neural spiking activity is possible through various complex mathematical models and simulations. The development of computational neuroscience has provided a detailed insight into spiking behaviors. Models like Integrate-and-Fire and FitzHugh–Nagumo simplify these dynamics to focus on the most critical aspects of neuron behavior. These models highlight the balance between excitation and inhibition, contributing to the study of neuronal synchronization and rhythm generation, which are fundamental to processes like perception and motor control.
Spiking Activity Patterns in Brain Cells
The brain contains a vast network of neurons, each displaying unique spiking activity patterns that reflect their role in processing information. Understanding these patterns provides insights into brain function and dysfunction.
- Synchronous Spiking: In certain cases, neurons can fire simultaneously, leading to synchronous patterns that are often observed during epilepsy.
- Oscillatory Patterns: Brain rhythms, such as alpha and gamma waves, arise from synchronized firing of neuronal populations, modulating cognitive processes.
- Poisson Process: Many neurons exhibit spiking patterns that resemble a Poisson distribution, indicating a variable yet somewhat predictable pattern of firing.
Neurons adapt their firing patterns based on input, a process that underpins learning and adaptation in the brain.
Spiking Activity in Neural Networks
Understanding spiking activity within neural networks is a core component of neuroscience. It integrates the electrical and chemical processes that facilitate neural communication. This activity is crucial for sensing and responding to the environment.
Importance of Spiking Activity in Neural Pathways
Spiking activity serves various functions in neural pathways, highlighting its critical role in the nervous system.
- Information Coding: Neurons encode and transmit information through the frequency and pattern of their spikes, which helps in processing sensory and motor information.
- Neural Synchronization: It aids in synchronizing the activity of ensembles of neurons, which is essential for coherent perception and movement.
- Learning and Memory: Spiking activity contributes to synaptic plasticity, a process crucial for learning and memory. This is exemplified by long-term potentiation, where repeated activation of synapses strengthens the connections.
- Dynamic Range: Neurons utilize spiking to maintain a broad dynamic range, allowing them to respond appropriately to varying input intensities.
In neuroscience, action potentials are often treated as all-or-nothing events, simplifying their complex physiological basis.
The synchronization of spiking activity underlies many rhythmic activities such as the circadian rhythms, which are synchronized to environmental cycles like light and dark. Neuronal circuits, through spiking, orchestrate these fundamental rhythms by synchronizing across different brain regions and levels of the nervous system. This orchestration is evident in phenomena such as brain waves, where groups of neurons fire synchronously, creating alpha, beta, and gamma waves, each corresponding to distinct cognitive and behavioral states.
Examples of Neural Networks Experiencing Spiking
Several examples illustrate the spiking activity within neural networks.
- Visual Cortex: Neurons in the visual cortex exhibit spiking patterns in response to visual stimuli, processing shapes, colors, and motion.
- Hippocampus: Known for its role in navigation and memory formation, the hippocampus displays place cell spiking patterns, which represent spatial locations.
- Basal Ganglia: Integral to motor control, spiking in this structure influences movement initiation and coordination.
- Auditory Cortex: Spiking in response to sound frequencies allows for the perception of pitch, rhythm, and speech.
An interesting example of spiking activity is observed in the somatosensory cortex, where neurons spike in response to tactile stimuli. When the skin is touched, sensory neurons relay this information through spikes, helping to discern texture, pressure, and vibration.
Spiking Activity Analysis Techniques
Analyzing spiking activity involves various techniques that provide insights into neural functioning. These techniques are pivotal for researchers seeking to understand the complex behaviors of neuronal networks.Methods for analyzing spiking activity are designed to capture the dynamics of neural firing patterns, which are essential for interpreting how the brain processes information.
Tools for Analyzing Spiking Activity
A range of tools and methodologies exist to help researchers analyze spiking activity effectively. These tools are fundamental for translating raw neural data into meaningful observations.The following are some of the notable tools used in this field:
- Electrophysiology: Techniques such as patch-clamp and multi-electrode arrays are used to record electrical activity from neurons. These methods help in detailing the patterns of spikes at a high temporal resolution.
- Statistical Analysis: Tools that apply statistical methods to assess spike train variability and correlations, enabling a deeper understanding of spiking behavior.
- Computational Models: Mathematical models simulate spiking activity, reproduce observed behaviors, and predict unmeasured phenomena. Common models used include the Hodgkin-Huxley and leaky integrate-and-fire models, which are described by equations like:\[C_m \frac{dV}{dt} = I_{Na} + I_K + I_L + I_{ext}\]
An example of analyzing spiking activity can be seen in the use of multi-electrode arrays to record neural activity in the visual cortex. Researchers can observe the synchronous firing of neurons in response to visual stimuli, providing insights into how visual information is processed in the brain.
Modern software tools, such as Spyke Viewer and NeuroExplorer, offer integrated platforms for analyzing and visualizing neural spike data.
Comparison of Analysis Methods
Different methods provide unique insights into spiking activity, each with its strengths and limitations. As researchers aim to study neural behaviors, selecting the appropriate analysis technique is crucial.
Method | Advantages | Disadvantages |
Electrophysiology | High temporal resolution, direct measurement of neuronal activity | Invasive, limited spatial coverage |
Optogenetics | High spatial resolution, ability to control specific neurons | Requires genetic manipulation, potential for photo-damage |
Computational Models | Ability to predict unobserved behaviors, low cost | Models require accurate parameters, can oversimplify complex dynamics |
A deeper look into computational models reveals their ability to integrate diverse data sources and predict complex dynamics that are challenging to observe directly. Models like the Izhikevich model blend biological realism and computational efficiency, replicating spike timings and neural patterns. These models are particularly useful when studying large-scale brain networks or simulating the effects of pathological changes, such as those seen in neurological disorders.
Causes of Irregular Spiking Activity in Neurons
Irregular spiking activity in neurons can have significant implications for normal brain function and is often associated with various neurological disorders. Understanding the factors that lead to such irregularities can provide insights into their potential effects on neural networks.
Factors Leading to Abnormal Spiking
Several factors can lead to abnormal spiking activity in neurons, disrupting normal neural communication and causing various forms of dysfunction.
- Genetic Mutations: Certain genetic mutations can alter the function of ion channels, leading to changes in the excitability of neurons and causing irregular spiking patterns.
- Neurotransmitter Imbalances: Imbalances in neurotransmitters, such as glutamate or GABA, can affect the excitation and inhibition balance, resulting in abnormal spike patterns.
- Environmental Influences: Factors such as toxins, drugs, and trauma can interfere with neural activity and lead to irregular spiking.
- Pathological Conditions: Disorders such as epilepsy, schizophrenia, and Alzheimer's disease are often associated with abnormal neuronal spiking.
In-depth studies have shown that irregular spiking activity often stems from the alteration of synaptic plasticity mechanisms. For instance, modifying the expression or function of receptors at the synapse can exacerbate the excitatory or inhibitory drive, thus affecting the overall network dynamics. Prolonged irregular activity can lead to compensatory changes, such as homeostatic plasticity, where neurons adjust their excitability to stabilize their activity over long periods. Investigating these compensatory mechanisms offers potential routes for therapeutic intervention in disorders characterized by irregular spiking.
Impact of Irregular Spiking on Neural Function
Irregular spiking activity can greatly impact various neural functions and behaviors, sometimes leading to significant health implications.
- Disrupted Communication: Abnormal spiking can impair synaptic communication, leading to issues in information processing and transmission across neural networks.
- Plasticity Changes: Altered spiking patterns can impact synaptic plasticity, hindering the brain's ability to learn and adapt.
- Behavioral Changes: Disorders like ADHD and autism, linked with irregular spiking, often exhibit behavioral changes and cognitive impairments.
- Pathogenesis of Disorders: Prolonged irregular spiking activity can contribute to the development and progression of neurological conditions like epilepsy and neurodegenerative disorders.
Advanced imaging and recording technologies, such as functional MRI and EEG, play crucial roles in observing the effects of irregular spiking on brain activity.
Consider a study where researchers use EEG to record brain activity in individuals with epilepsy. They observe distinct irregular spiking patterns that correspond with seizure events, highlighting the critical link between abnormal spiking and the disorder's symptoms.
spiking activity - Key takeaways
- Definition of Spiking Activity in Neurons: It refers to the firing of neurons as electrical signals known as action potentials.
- Spiking Activity Analysis Techniques: Methods such as electrophysiology, statistical analysis, and computational models are used to analyze neural firing patterns.
- Spiking Activity in Neural Networks: Spiking is crucial for communication in neural networks, affecting processes like sensing, movement, learning, and memory.
- Characteristics of Neural Spiking Activity: Includes frequency, amplitude, refractory period, and temporal patterns, influencing information encoding and transmission.
- Spiking Activity Patterns in Brain Cells: Patterns such as synchronous and oscillatory patterns offer insights into neural function and brain processes.
- Causes of Irregular Spiking Activity in Neurons: Factors such as genetic mutations, neurotransmitter imbalances, environmental influences, and pathological conditions contribute to irregular spiking.
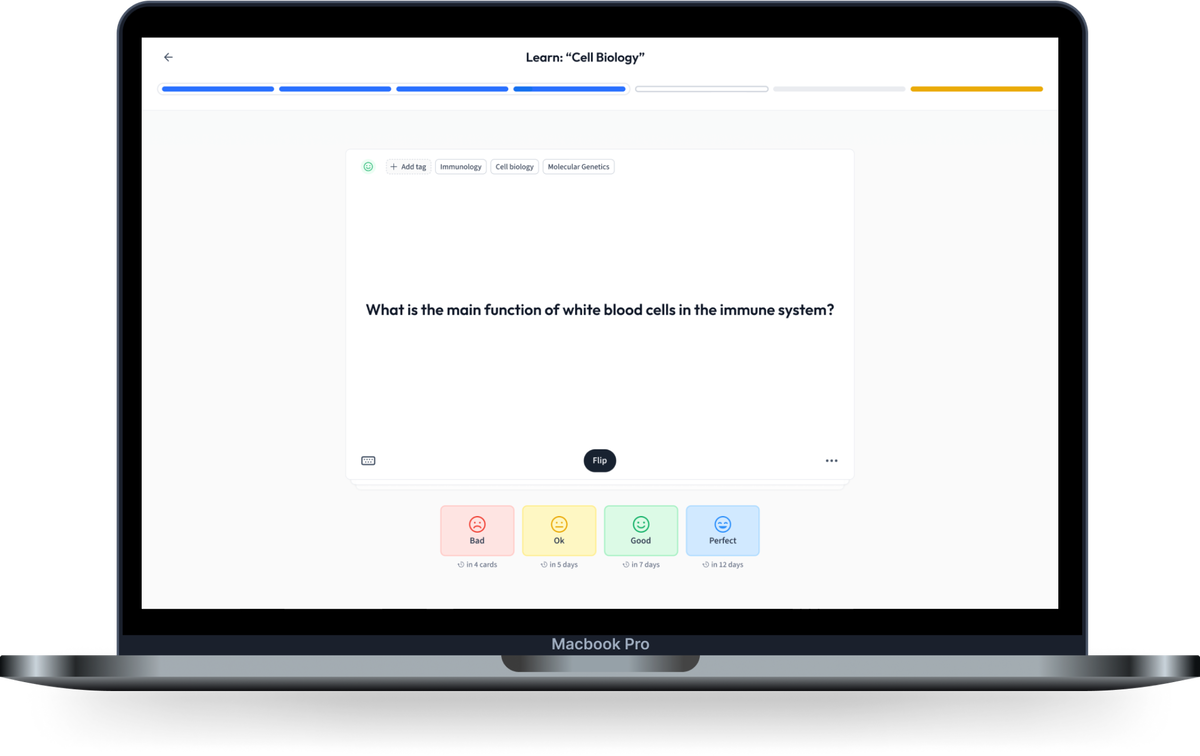
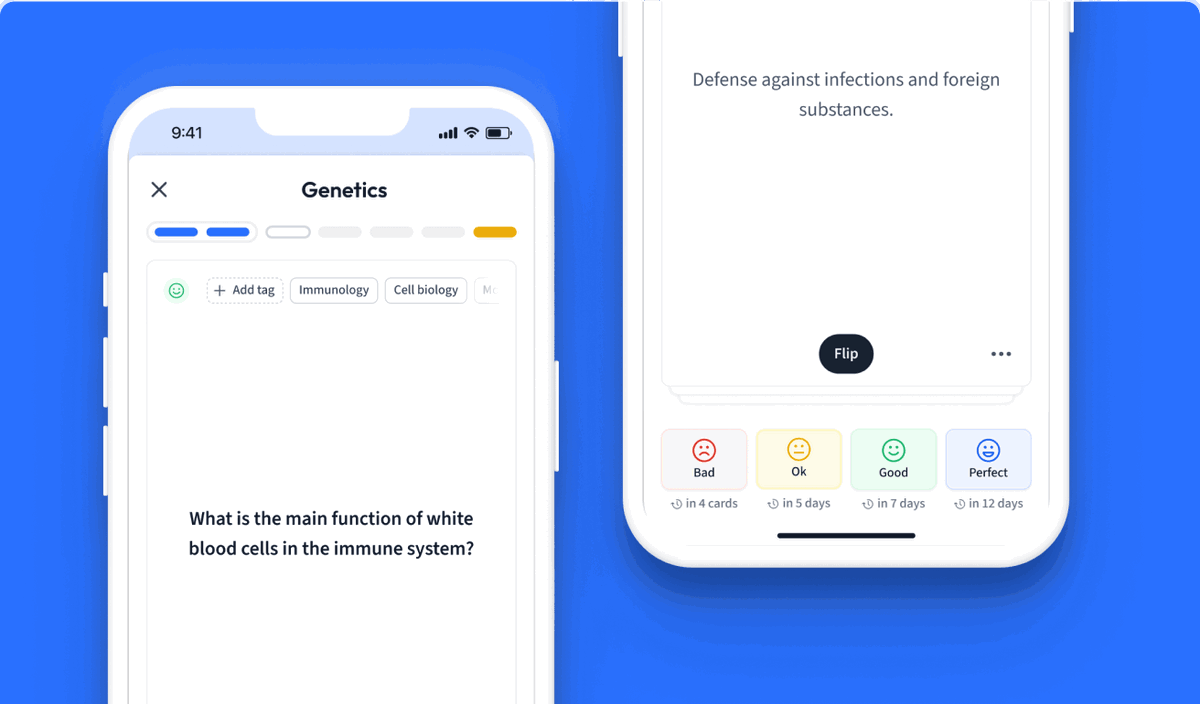
Learn with 12 spiking activity flashcards in the free StudySmarter app
Already have an account? Log in
Frequently Asked Questions about spiking activity
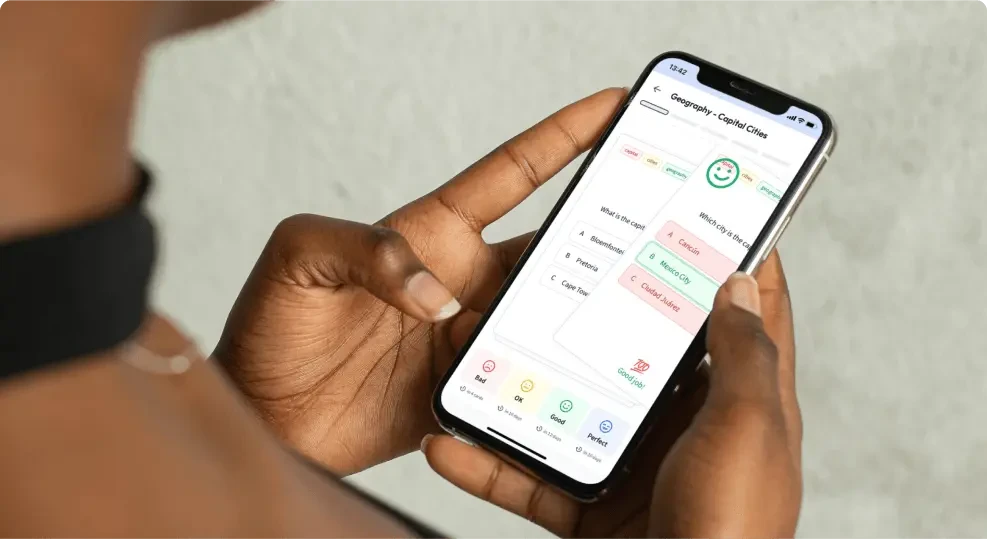
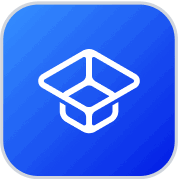
About StudySmarter
StudySmarter is a globally recognized educational technology company, offering a holistic learning platform designed for students of all ages and educational levels. Our platform provides learning support for a wide range of subjects, including STEM, Social Sciences, and Languages and also helps students to successfully master various tests and exams worldwide, such as GCSE, A Level, SAT, ACT, Abitur, and more. We offer an extensive library of learning materials, including interactive flashcards, comprehensive textbook solutions, and detailed explanations. The cutting-edge technology and tools we provide help students create their own learning materials. StudySmarter’s content is not only expert-verified but also regularly updated to ensure accuracy and relevance.
Learn more