Jump to a key chapter
Introduction to Nanotechnology in Oncology
Nanotechnology is revolutionizing the field of oncology, offering novel solutions for the diagnosis and treatment of cancer. By manipulating materials at the atomic and molecular levels, it presents opportunities to improve how cancer is detected, monitored, and combated. This field is rapidly advancing, integrating with existing medical technologies to provide enhanced precision and effectiveness in cancer care.As you explore this fascinating topic, you will uncover how nanotechnology can potentially change the landscape of oncology, benefiting patients and medical practitioners alike.
Definition of Nanotechnology in Oncology
Nanotechnology in Oncology refers to the application of nanotechnology in the diagnosis and treatment of cancer. It involves the use of nanoparticles, which are particles between 1 and 100 nanometers in size, to target cancer cells with high precision while minimizing damage to healthy tissues.
This innovative approach leverages the unique properties of nanoparticles to deliver drugs more effectively, enhance imaging techniques, and even directly target cancer cells for destruction. Components of this technology include:
- Nanoparticles: Engineered for specific tasks such as targeting cancer cells or delivering chemotherapy drugs selectively.
- Nanosensors: Enhance the detection of cancer markers in the body, aiding early diagnosis.
- Nanoshells and Nanotubes: Used in thermal therapies to destroy tumors by converting light energy into heat.
Imagine nanoparticles as tiny couriers carrying medicine. In chemotherapy, instead of affecting both cancerous and healthy cells, the nanoparticles deliver the drugs directly to the cancer cells, reducing side effects and enhancing treatment efficacy.
Nanotechnology allows the development of smart particles that can change behavior in response to external stimuli, such as changing shape when reaching a tumor site.
Historical Background of Nanotechnology in Oncology
The journey of nanotechnology in oncology began several decades ago, evolving with significant advancements in the field of material science and cancer research. The integration of nanotechnology in medical applications gained momentum in the 1980s with the development of specialized nanoparticles designed for drug delivery and imaging. By the 2000s, research began focusing more intensely on utilizing nanoparticles for specific cancer treatments.Key developments in the historical timeline include:
Year | Milestone |
1987 | Introduction of liposome-based drug delivery systems. |
1995 | Nasal vaccines created using nanoparticles. |
2005 | Smart drug delivery systems began to emerge. |
2010 | Application of gold nanoparticles in thermal ablation, a techique to destroy cancer cells with heat. |
In the recent historical context of nanotechnology in oncology, quantum dots have made special contributions to the improvement of imaging techniques. Quantum dots are semiconductor particles that, due to their quantum mechanical properties, can fluoresce brightly and in a range of colors. This property is utilized in imaging applications, enabling doctors to visualize cancerous tissues with more precision than traditional contrast agents. This innovation significantly enhances the ability to spot tumors during early stages, potentially improving treatment outcomes. Quantum dots are also explored for their use in multiplexing, which allows simultaneous tracking of different biological processes in a single session, providing a comprehensive picture of cancer progress or response to treatment.
Use of Nanotechnology in Oncology
The integration of nanotechnology in oncology marks a significant leap forward in the field of cancer treatment and diagnosis. By utilizing materials at the nanoscale, this technology offers new ways to treat cancer more effectively while minimizing harm to healthy tissues. This emerging approach promises to enhance precision and reduce side effects, revolutionizing how cancer is managed.
Current Applications of Nanotechnology in Cancer Therapy
Nanotechnology in cancer therapy has led to numerous innovative applications that are being explored and implemented in clinical settings. These applications include both the treatment and diagnosis of cancer, improving outcomes for patients. Current applications include:
- Targeted Drug Delivery: Nanoparticles can carry chemotherapy drugs directly to cancer cells, reducing systemic toxicity.
- Imaging Enhancements: Nanocarriers improve the visualization of tumors through advanced imaging techniques.
- Thermal Therapy: Nanoparticles can convert external energy sources, such as light, into heat that destroys cancer cells.
- Gene Therapy: Nanoscale delivery systems carry genetic material that can alter the behavior of cancer cells.
Consider a nanoparticle designed to deliver paclitaxel, a common chemotherapy drug. When modified to release the drug only upon reaching a tumor cell, these particles can significantly reduce collateral damage to normal cells, enhancing patient recovery and outcomes.
Current research indicates that the use of iron oxide nanoparticles can not only enhance MRI imaging but also be used in hyperthermia, where they heat up to kill tumor cells without affecting surrounding tissues.
Mathematical modeling plays a crucial role in optimizing the design and function of nanoparticles. Understanding how these particles behave in biological systems can greatly enhance their effectiveness. For instance, calculating the optimal dose and release rate involves sophisticated equations. One such relation for drug release kinetics might be modeled using the following equation: \[ \frac{dC}{dt} = -k \times C^n \] where \( C \) is the concentration of the drug within the nanoparticle, \( t \) is time, \( k \) is the rate constant, and \( n \) is the release order. This equation is essential for predicting how long it will take for a nanoparticle to release its full dosage once inside a tumor, ensuring timely and effective treatment.
Role of Nanoparticles in Cancer Therapy
Nanoparticles are the cornerstone of nanotechnology in oncology. These tiny particles can be engineered to fulfill specific roles in cancer therapy, from drug delivery to assisting in diagnostics. They excel at providing treatments that are not only effective but also limit adverse side effects. Here’s how they function:
- Target Specificity: Nanoparticles can be designed with ligands on their surface that bind to receptors on cancer cells, allowing for precise targeting.
- Controlled Release: These particles can be programmed to release their therapeutic payload at a controlled rate, maximizing efficacy.
- Biocompatibility: Nanoparticles are designed to be safe and acceptable to the body, reducing immune reactions.
Target Specificity is the ability of nanoparticles to seek out and bind to cancer cells with minimal impact on healthy cells, using molecular markers that are specific to cancer cells.
To ensure maximum effect, nanoparticles are often engineered to be multifunctional, addressing several therapeutic pathways simultaneously.
Techniques of Nanotechnology in Cancer Treatment
Nanotechnology offers cutting-edge techniques for cancer treatment, focusing on precision and minimal side effects. By incorporating nanotechnology, the efficacy of cancer treatments can be significantly enhanced, providing patients with improved outcomes. Let's explore these innovative approaches and how they compare to traditional treatments.
Innovative Techniques in Nanotechnology for Cancer
Several innovative techniques utilizing nanotechnology have emerged in recent years, providing more targeted and effective cancer therapies. These techniques harness the unique properties of nanoparticles to improve the delivery and efficacy of cancer treatments.Some prominent techniques include:
- Nanoparticle Drug Delivery Systems: By carrying chemotherapy drugs directly to cancer cells, these systems minimize side effects and enhance treatment efficiency.
- Photothermal Therapy: Nanoparticles absorb light and convert it into heat, selectively destroying cancer cells while sparing healthy tissue.
- Nucleic Acid-Based Nanocarriers: These deliver genetic material to modify or silence cancerous genes, offering a novel approach to cancer treatment.
For instance, liposomes, a type of nanoparticle, have been used to deliver doxorubicin, an anthracycline chemotherapy drug. By encapsulating the drug in nanoscale particles, the delivery is targeted directly to tumor cells, reducing the cardiotoxicity often associated with doxorubicin treatment.
One of the most intriguing aspects of nanotechnology in cancer treatment is the use of quantum dots for early detection and imaging. Quantum dots are nanoscale semiconductor particles that can emit light when stimulated. Because of their precise light-emitting capabilities, they are ideal for imaging applications, providing clearer contrast than traditional dyes. When applied in oncology, these particles can bind to cancer cells, allowing for high-resolution imaging that identifies tumors at earlier stages. This capability can significantly enhance the early detection of cancers, ensuring treatments begin sooner when they are most effective.
Comparison of Traditional and Nanotechnology-Based Treatments
When comparing traditional cancer treatments, such as chemotherapy and radiation, with nanotechnology-based methods, several differences and advantages become apparent.Traditional methods often affect healthy and cancerous cells alike, leading to significant side effects. In contrast, nanotechnology aims to increase the precision of treatment, minimizing impact on surrounding tissues. The following table outlines key differences between these approaches:
Treatment Type | Traditional | Nanotechnology-Based |
Targeting | Non-specific | Highly specific |
Side Effects | High | Lower due to targeted delivery |
Efficiency | Variable | Increased with enhanced targeting |
Innovation Level | Established | Rapidly evolving |
Nanotechnology holds the potential to significantly reduce the occurrence of drug resistance by maintaining therapeutic drug levels precisely at the cancer site.
Advantages of Nanotechnology in Oncology
Nanotechnology offers several pioneering methods to enhance cancer treatment, significantly different from traditional approaches. The precision of these methods brings numerous advantages to oncology that extend from improved drug delivery systems to targeted therapy.
Improved Drug Delivery with Nanotechnology
One of the most notable benefits of nanotechnology in oncology is its ability to improve drug delivery. By employing nanoparticles, precise delivery of therapeutic agents directly to cancer cells is achievable, greatly reducing systemic side effects.
- Enhanced Targeting: Nanoparticles can home in on cancer cells, sparing healthy tissues from exposure.
- Controlled Release: They enable controlled and sustained release of drugs, maintaining effective concentrations at the tumor site over time.
- Stability and Solubility: Nanoparticles can improve the solubility and stability of drugs, enhancing their bioavailability.
Consider a nanoparticle encapsulating doxorubicin, a chemotherapy drug. The nanoparticle system can be engineered to release the drug when encountering acidic environments typical to tumor sites, thus maximizing its therapeutic effects specifically where needed.
Nanoparticles can be multifunctional, carrying both therapeutic drugs and diagnostic agents, allowing for simultaneous treatment and monitoring of cancer.
The mathematics behind the drug release from nanoparticles is modeled using several equations. A common model is the Higuchi equation, which describes the drug release rate from a solid matrix: \[ Q = A \times D \times \frac{(2C_0 - C_s)t}{2L} \]where \( Q \) is the cumulative amount of drug released, \( A \) is the surface area of the nanoparticle, \( D \) is the diffusion coefficient of the drug in the matrix, \( C_0 \) is the initial drug concentration, \( C_s \) is the solubility of the drug, \( t \) is time, and \( L \) is the thickness of the diffusion layer.By using such detailed mathematical models, researchers can predict how long a drug will release from its nanoparticle carrier under various conditions, optimizing the formulation for maximal efficacy. This precision allows for more effective treatment regimens tailored to individual patients' needs.
Advantages of Nanoparticles in Targeted Therapy
Nanoparticles offer distinct advantages in targeted therapy, focusing treatment on cancerous cells while avoiding healthy ones. This method revolutionizes cancer treatment by increasing its precision and reducing side effects.
- Specificity: Nanoparticles can be designed to bind specific cancer cell receptors, ensuring that therapeutic agents reach only the target cells.
- Reduced Dosage: By efficiently delivering drugs directly to cancer cells, the overall dose required can be reduced, decreasing harmful side effects.
- Multifunctionality: They can combine therapeutic and diagnostic functions, serving as both treatment providers and disease trackers.
Utilizing folic acid-modified nanoparticles can exploit the high expression of folate receptors on certain cancer cells. This property enhances the selective uptake of medicated nanoparticles by cancer cells, improving treatment outcomes.
Specificity in this context refers to the ability of nanoparticles to selectively interact with cancer cells, minimizing interactions with non-targeted cells.
In targeted therapy, nanoparticles can also carry genes or RNA molecules that can disrupt cancer cell processes, representing a new frontier in gene therapy.
Understanding the behavior of nanoparticles in targeting therapy is enhanced by mathematical modeling. One such model involves calculating the binding affinity of nanoparticles with cancer cell receptors, often described by the Langmuir adsorption isotherm: \[ \theta = \frac{KL[C]}{1 + KL[C]} \] where \( \theta \) is the surface coverage, \( K \) is the equilibrium constant, \( L \) is a factor related to the amplification of signal in specific conditions, and \([C]\) is the concentration of receptor-ligand complexes.This equation helps predict how well nanoparticles will bind to cancer cells, guiding the design and application of nanoparticle-based medicines for optimal targeting effectiveness. Models like this one are crucial for understanding and improving how these nanoparticles interact with biological systems, ensuring that therapies are both safe and potent.
Future of Nanotechnology in Oncology
The future of nanotechnology in oncology is poised to bring transformative developments to cancer treatment and diagnosis. As research advances, new trends and anticipated developments will further enhance the precision, effectiveness, and accessibility of cancer therapies.
Emerging Trends in Nanotechnology for Cancer Treatment
In the field of oncology, nanotechnology is continuously evolving, with several emerging trends that promise to improve cancer treatment outcomes. These trends leverage the unique properties of nanoparticles to advance cancer therapies and diagnostics.
- Enhanced Imaging Techniques: Nanoparticles improve contrast in imaging, allowing for earlier and more accurate detection of tumors.
- Combination Therapies: Utilizing nanoparticles to deliver multiple drugs simultaneously for synergistic effects.
- Personalized Medicine: Tailoring nanoparticle therapies to individual genetic profiles to optimize efficacy.
Nanoparticles can be engineered to release drugs in response to specific environmental triggers, such as temperature or pH changes, at the tumor site.
Consider a scenario where magnetic nanoparticles are employed to enhance MRI scans. These particles improve the resolution of images, helping clinicians to determine the precise size and location of a tumor, which is crucial for planning effective treatment strategies.
A sophisticated development is the use of biodegradable nanoparticles in targeted cancer therapy. These nanoparticles are designed to decompose into non-toxic byproducts after delivering their therapeutic payload, reducing the long-term burden on the patient's system. The degradation process can be controlled by altering the chemical structure of the nanoparticles. For example, the rate at which a polymer nanoparticle degrades can be described by the equation: \[ M_t/M_\infty = k \times t^n \]where \( M_t/M_\infty \) is the fraction of polymer mass remaining at time \( t \), \( k \) is the degradation rate constant, and \( n \) denotes the degradation order. By adjusting \( k \) and \( n \), scientists can design nanoparticles to degrade at an optimal rate, releasing drugs precisely when and where they are needed, exemplifying the nuances of this technology in practical applications.
Anticipated Developments in Nanotechnology and Oncology
The advancements in nanotechnology hold promising potential for the future of oncology, marking significant developments in the fight against cancer. Key anticipated developments include:
- Smart Drug Delivery Systems: Utilizing stimuli-responsive nanoparticles that can adapt their behavior according to the biological environment.
- Nano-vaccines: Creating vaccines at the nanoscale for preventing cancer by harnessing the body’s immune response.
- Advanced Therapeutic Modalities: Leveraging nanotechnology for novel therapies, such as photodynamic therapy or gene editing, aimed at cancer cells.
Future nanoparticles might have self-destruct mechanisms, where they safely break down after performing their function, to minimize long-term impacts on the body.
Exploring the genetic aspects of cancer treatment is a frontier in nanotechnology, with nanoparticle-based systems poised to deliver gene-editing tools like CRISPR-Cas9. This approach could allow for precise corrections of genetic abnormalities within cancer cells, reframing treatment strategies. The mechanics of this can be exemplified by considering the probability that a nanoparticle successfully delivers its CRISPR payload to a targeted gene site, potentially modeled by the binomial probability formula:\[ P(X = k) = \binom{n}{k}p^k(1-p)^{n-k} \]where \( n \) is the total number of trials, \( k \) is the number of successful deliveries, and \( p \) is the probability of success on an individual trial. Understanding and manipulating these probabilities will be essential for optimizing the therapeutic efficacy of gene-editing nanoparticles, bringing precision medicine to the forefront of oncology.
nanotechnology in oncology - Key takeaways
- Nanotechnology in Oncology: Refers to using nanotechnology for cancer diagnosis and treatment, involving nanoparticles to target cancer cells precisely.
- Role of Nanoparticles in Cancer Therapy: Nanoparticles are engineered for specific cancer therapy tasks like targeted drug delivery, controlled release, and improving imaging techniques.
- Application of Nanotechnology in Cancer Therapy: Includes targeted drug delivery, improved imaging, thermal therapy, and gene therapy using nanocarriers.
- Advantages of Nanotechnology in Oncology: Enhanced precision in treatment and diagnosis, reduced side effects, and improved drug delivery by targeting cancer cells specifically.
- Techniques of Nanotechnology in Cancer Treatment: Involves nanoparticle drug delivery systems, photothermal therapy, and nucleic acid-based carriers for targeted treatment.
- Future of Nanotechnology in Oncology: Emerging trends include smart drug delivery systems, personalized medicine, and advanced imaging techniques for more precise cancer therapies.
Learn faster with the 10 flashcards about nanotechnology in oncology
Sign up for free to gain access to all our flashcards.
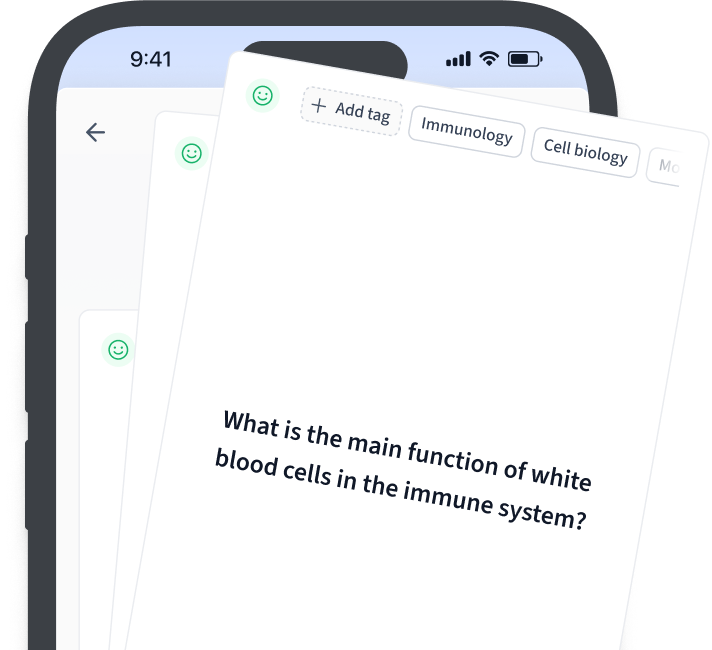
Frequently Asked Questions about nanotechnology in oncology
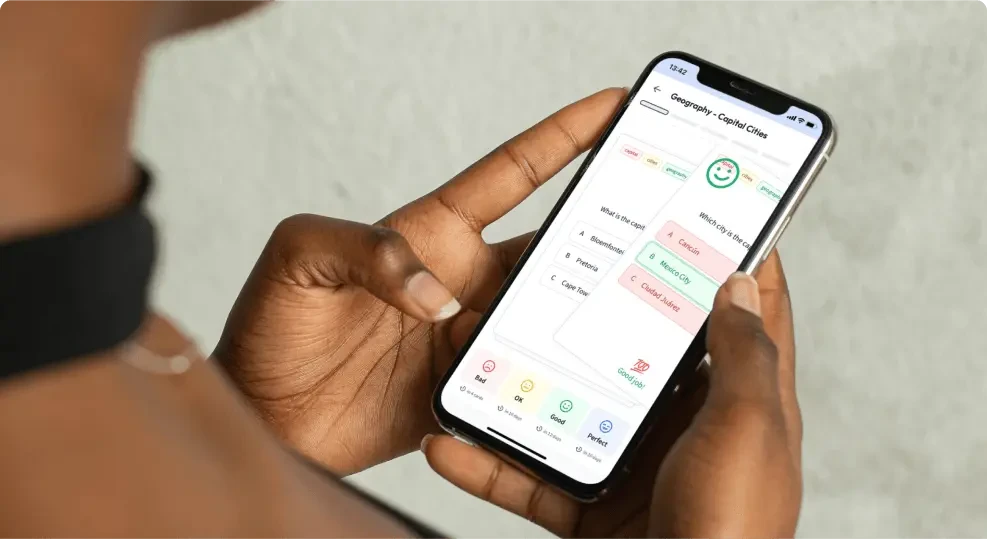
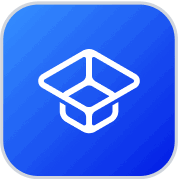
About StudySmarter
StudySmarter is a globally recognized educational technology company, offering a holistic learning platform designed for students of all ages and educational levels. Our platform provides learning support for a wide range of subjects, including STEM, Social Sciences, and Languages and also helps students to successfully master various tests and exams worldwide, such as GCSE, A Level, SAT, ACT, Abitur, and more. We offer an extensive library of learning materials, including interactive flashcards, comprehensive textbook solutions, and detailed explanations. The cutting-edge technology and tools we provide help students create their own learning materials. StudySmarter’s content is not only expert-verified but also regularly updated to ensure accuracy and relevance.
Learn more