Jump to a key chapter
Astroecological Systems Overview
Astroecological systems are complex and fascinating. They refer to ecosystems that exist in outer space environments or are involved in space exploration and habitation.
Understanding Astroecological Systems
In studying astroecological systems, you learn about the interaction between living organisms and their extraterrestrial environment. This field combines several disciplines including biology, ecology, astronomy, and physics to examine how life can sustain itself beyond Earth.
An astroecological system refers to the ecological interactions that occur in outer space environments, or in habitats designed for sustaining life outside Earth.
The concept of astroecology includes considering how life could potentially influence planetary processes in other worlds.
Example: A space station with a self-sustaining biosphere is an example of an astroecological system. It requires careful balance of light, nutrients, and water to support plant growth and human life.
Mathematical Modelling in Astroecological Systems
Mathematical models play a crucial role in understanding astroecological systems. These models help predict how ecosystems can maintain balance in outer space. Consider the basic population model for plant growth in a space habitat, which can be represented by the logistic growth equation: \[P(t) = \frac{K}{1+\frac{K-P_0}{P_0}e^{-rt}}\]In this formula:
- P(t) is the population at time t.
- K is the carrying capacity of the environment.
- P_0 is the initial population.
- r is the growth rate.
Deep Dive: Consider the nutrient cycle in a Martian habitat. The nitrogen cycle, an essential part of maintaining plant life, could hypothetically be modeled and sustained in a Martian habitat by using soil brought from Earth or synthesized from Martian materials. Enabling a stable nitrogen cycle would require conversion of inert nitrogen to biologically useful forms. Microorganisms in engineered biospheres could convert atmospheric nitrogen (N₂) into ammonia (NH₃) via nitrogen fixation, introducing it into the ecosystem. This could involve careful infrastructure design, monitoring plant and microbial health, and dynamic adjustments to environmental conditions to simulate Earth's balanced ecosystem.
Physics of Ecosystems in Space
Exploring the physics behind ecosystems in space involves understanding unique interactions between physical laws and biological processes. These interactions occur within specially designed habitats or potential planetary environments beyond Earth.
Components of Space Ecosystems
Space ecosystems consist of several essential components that interact to sustain life. These include:
- Atmosphere: A controlled mixture of gases suitable for respiration.
- Living Organisms: Plants, microbes, and possibly animals that depend on and contribute to the ecosystem.
- Water: An essential resource for hydration, metabolic processes, and nutrient transport.
- Nutrients: Such as nitrogen and phosphorus, required for life processes.
- Energy Source: Typically artificial lighting or solar energy to support photosynthesis and other processes.
Example: Imagine a closed-loop hydroponic system aboard a spacecraft where plants grow without soil. Water and nutrients circulate among plant roots, and their growth is fueled by LED lights mimicking sunlight. This environment forms a basic space ecosystem.
A closed-loop system in space ecosystems refers to a self-sustaining cycle where resources are continually recycled and reused within the habitat.
Interactions in Astroecological Systems
Within astroecological systems, interactions follow strict physical laws, often involving:
- Thermodynamic Processes: Energy exchanges, like photosynthesis, follow principles like conservation of energy.
- Mass Transfer: Movement of nutrients and water is governed by mass conservation and transport equations.
- Gravity: Reduced gravity impacts fluid behavior and biological functions.
Deep Dive: Consider how microgravity affects plant growth. In outer space, plants experience altered cellular signal transduction and mechanical stress due to different gravity conditions. Research in microgravity observes changes in gene expression related to growth, enabling better understanding of how plants adapt to space's unique environments. Such studies benefit future space agriculture, crucial for sustaining human colonies on other planets.
Gravity levels on Mars are about 38% of those on Earth, affecting potential astroecological systems designed for Mars settlements.
Evolution of Space Ecosystems
Space ecosystems evolve over time through complex dynamics shaped by environmental conditions and biological adaptation. This evolution can be modeled to predict future shifts and help design resilient systems. Key factors influencing this evolution include:
- Mutation and Selection: Genetic changes and natural selection can lead to adaptation in living organisms.
- Environmental Fluctuations: Changes in conditions, like radiation levels, impact ecosystem stability.
- Human Intervention: Modifications by astronauts or researchers affect ecosystem evolution.
N | Population size |
t | Time |
r | Intrinsic growth rate |
K | Carrying capacity |
Astroecology and Its Importance
Astroecology is an interdisciplinary field that examines how ecosystems operate in extraterrestrial environments. It bridges biology, ecology, and physics to analyze how life can thrive beyond Earth's boundaries and is crucial in advancing our understanding of sustainable life in space.
Understanding Astroecological Models
Astroecological models are essential tools that simulate extraterrestrial ecosystems. These models integrate mathematical equations to predict and manage ecosystem dynamics in space habitats.
An astroecological model is a conceptual and mathematical representation that predicts the behavior of an extraterrestrial ecosystem.
Consider a model representing plant growth in a Mars-based habitat, where population dynamics can be defined by the equation:\[P(t) = P_0 e^{rt}\]Here:
- \boldsymbol{P(t)} = Population at time t
- \boldsymbol{P_0} = Initial population
- \boldsymbol{r} = Growth rate
Example: Consider the simulation of nutrient exchange between plants and soil microbes inside a closed habitat on the Moon. Using such astroecological models helps predict how nutrients recycle effectively, influencing food production systems for lunar bases.
Deep Dive: Delving into microgravity effects on plant root structures, models show altered growth patterns due to changes in gravitational pull. Without the downward force felt on Earth, roots may spread differently, impacting nutrient uptake and growth efficiency. Understanding these models informs the development of plant-support systems optimized for space environments.
Applications of Astroecological Systems
The applications of astroecological systems are diverse, playing a role in the design of life-support systems for space exploration and potential habitation on extraterrestrial bodies.
A life-support system refers to a mechanism or series of processes designed to maintain all necessary conditions for human life in space, often incorporating astroecological principles.
Astroecological systems support:
- Spacecraft biospheres: Recycling air, water, and waste effectively for long missions.
- Lunar and Martian Bases: Providing sustainable resources for colonies.
- Agricultural Development: Growing food in microgravity and extreme conditions.
Example: On long-duration missions to Mars, astroecological systems can create self-reliant biospheres, minimizing the need for supply transports from Earth. With closed-loop systems, astronauts could produce all of their oxygen, water, and food.
Researchers are investigating artificial gravity solutions to integrate with astroecological systems, leveraging centrifugal force to mimic Earth's gravity in rotating habitats.
Role of Astroecological Systems in Space Exploration
Astroecological systems are pivotal in current and future space exploration missions, aiding in sustainable space habitation and the possibility of interplanetary colonization.
Such systems play vital roles including:
- Supporting long-term missions: By maintaining biospheres that adapt to space conditions.
- Enabling extraterrestrial agriculture: Through sustainable growth systems.
- Aiding scientific research: By providing data on how life can sustain in varying space conditions.
Deep Dive: Future astroecological systems may incorporate AI to optimize resource management dynamically, monitoring plant health, nutrient levels, and environmental conditions in real-time. Predictive models enhanced by machine learning could adjust parameters to ensure optimal conditions for life, which is crucial for autonomous operations on distant planets or moons.
Astroecological Models and Equations
Astroecological models and their associated equations are crucial for understanding how ecosystems can sustain themselves beyond Earth. These sophisticated models help scientists predict the dynamics of life-supporting environments in extraterrestrial conditions.
Development of Astroecological Models
The development of astroecological models is a complex process that involves combining insights from biology, physics, and environmental science. Significant steps include:
- Identifying key ecosystem components needed for sustainability.
- Integrating interdisciplinary data from experiments and observations.
- Using computational tools to simulate ecosystem dynamics.
- P is the production rate of oxygen through photosynthesis.
- R is the respiration rate of organisms consuming oxygen.
- C is any oxygen loss by chemical reactions or leakage.
An astroecological model is a conceptual and mathematical model depicting the interactions, sustainability, and behavior of ecosystems in extraterrestrial environments.
Example: One example of an astroecological model is the Biosphere 2 project, which sought to replicate Earth's ecosystem within a sealed structure to study self-sustaining habitats. This project provided valuable data on resource cycles, species interactions, and sustainability challenges.
Advanced astroecological models may incorporate real-time monitoring and machine learning algorithms for predictive adaptations.
Key Astroecological Equations
Key equations in astroecology are fundamental tools that express the relationships between various components of space ecosystems. They aid in designing efficient life-support systems by quantifying ecosystem dynamics. Notable examples include the logistic growth equation for predicting population dynamics, expressed as:\[P(t) = \frac{K}{1+\frac{K-P_0}{P_0}e^{-rt}}\]Where:
- P(t) is the population at time t.
- K is the carrying capacity.
- P_0 is the initial population.
- r is the intrinsic growth rate.
Deep Dive: Consider how thermodynamic laws apply to closed-loop ecological systems. In space habitats, recycling resources through energy-intensive processes must comply with the laws of thermodynamics. This involves calculating energy efficiency and losses, incorporating heat transfer models to optimize resource use, and maintaining stability over long durations. Such considerations are pivotal in designing self-sustaining life systems for deep space missions.
Predictive Power of Astroecological Models
The predictive power of astroecological models lies in their ability to forecast ecosystem behavior under various scenarios, which include:
- Environmental stress factors, like radiation or temperature changes.
- Resource scarcity, such as limited water supply.
- Biotic interactions, including predator-prey dynamics.
- I is the nutrient input from sources like recycled waste.
- O is the nutrient output via plant uptake.
- D is the degradation or loss through system inefficiencies.
Example: By employing astroecological models, scientists predict algae growth patterns used for oxygen production and waste recycling in spacecraft. This helps optimize environmental conditions and resource use during long missions.
Astroecological models can be continually refined with data from space missions, ensuring adaptability to real-world conditions.
astroecological systems - Key takeaways
- Astroecological Systems: Ecosystems that exist or are designed for outer space environments or exploration.
- Physics of Ecosystems in Space: Study of interactions between physical laws and biological processes in space habitats.
- Astroecology: Interdisciplinary field examining ecosystem operations in extraterrestrial environments, combining biology, ecology, and physics.
- Astroecological Models: Conceptual and mathematical tools simulating extraterrestrial ecosystem behavior to predict and manage space habitats.
- Astroecological Equations: Mathematical expressions used in models to describe ecosystem dynamics, such as the logistic growth equation.
- Space Ecosystems: Components like atmosphere, living organisms, water, and energy sources that interact in designed habitats to sustain life.
Learn faster with the 12 flashcards about astroecological systems
Sign up for free to gain access to all our flashcards.
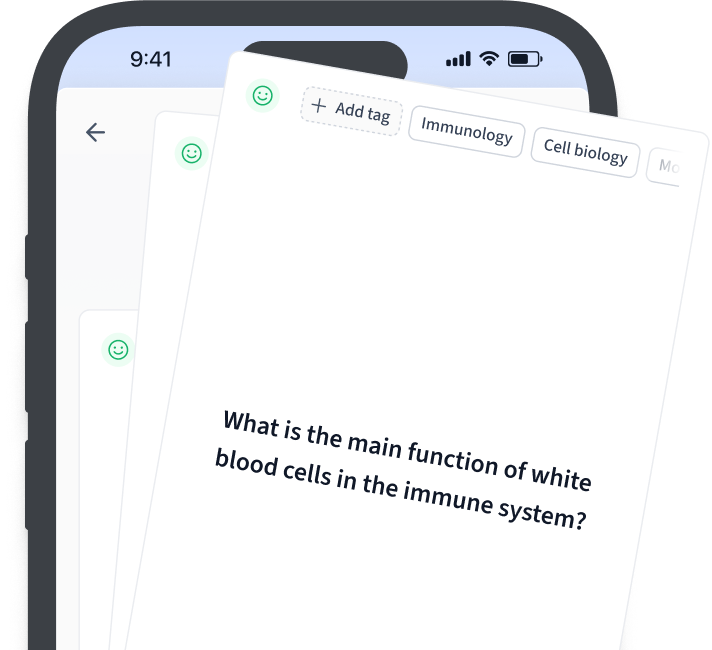
Frequently Asked Questions about astroecological systems
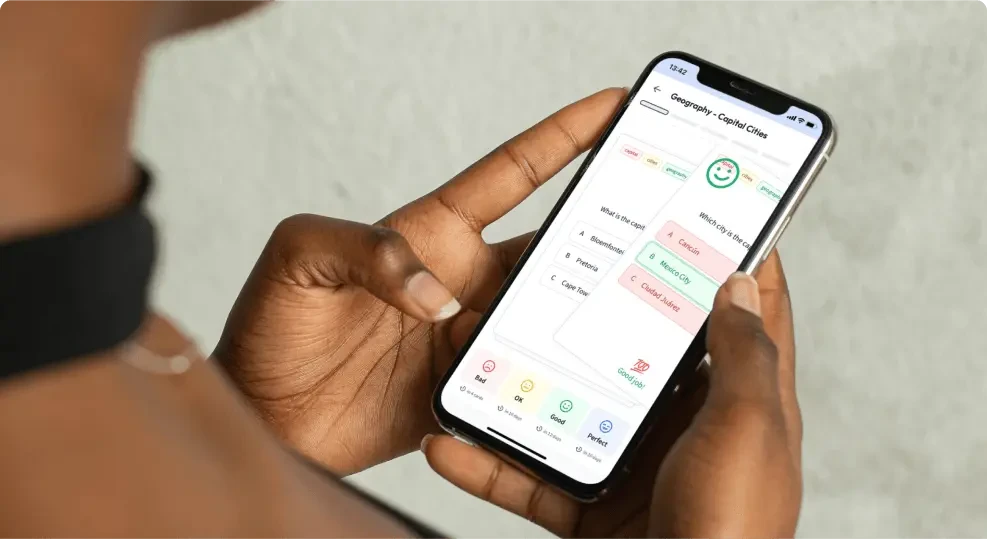
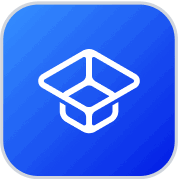
About StudySmarter
StudySmarter is a globally recognized educational technology company, offering a holistic learning platform designed for students of all ages and educational levels. Our platform provides learning support for a wide range of subjects, including STEM, Social Sciences, and Languages and also helps students to successfully master various tests and exams worldwide, such as GCSE, A Level, SAT, ACT, Abitur, and more. We offer an extensive library of learning materials, including interactive flashcards, comprehensive textbook solutions, and detailed explanations. The cutting-edge technology and tools we provide help students create their own learning materials. StudySmarter’s content is not only expert-verified but also regularly updated to ensure accuracy and relevance.
Learn more