Jump to a key chapter
Brightness Temperature Definition Physics
Exploring the concept of brightness temperature is essential for understanding how different celestial objects emit radiation. This term captures the comparison of the observed intensity of radiation with that from a black body — an idealized physical body that absorbs all incident electromagnetic radiation.
The brightness temperature is defined as the temperature a black body would need to have to emit the same amount of radiation at a specific frequency. Mathematically, it can be calculated using Planck's Law for black body radiation.
Understanding Brightness Temperature
Brightness temperature helps in determining the properties of an object or surface by analyzing the radiation it emits. Since no real object perfectly emits or absorbs radiation like a black body, brightness temperature is often higher than the physical temperature.
In radio astronomy, brightness temperature is a crucial parameter. As it's difficult to measure the actual temperature of distant stars and galaxies, their emitted radio waves are analyzed. By approximating the brightness temperature, scientists can make inferences about astronomical phenomena. Interestingly, the cosmic microwave background radiation has a brightness temperature of about 2.7 K, giving insights into the early universe.
An example of using brightness temperature in practical applications is the observation of the Sun. The Sun's photosphere has a brightness temperature of approximately 5800 K. This measurement helps in understanding its energy output and structure.
Remember that brightness temperature often differs from the actual physical temperature because it depends on emission and absorption properties.
Calculating Brightness Temperature
To find the brightness temperature of an object, you can use Planck's Law in its simplified form for radio wavelengths. The formula is given by:\[ B(T) = \frac{2kT}{\lambda^2} \]where:
- B(T) is the brightness at temperature T,
- k is the Boltzmann constant,
- T is the absolute temperature,
- \( \lambda \) is the wavelength.
Brightness Temperature Formulas and Calculations
When calculating the brightness temperature, you are essentially comparing the amount of radiation emitted by an object to that emitted by a black body. Here, we'll explore the formulas and specific steps required for these calculations.
Key Formula for Brightness Temperature
The core formula for determining brightness temperature requires knowledge of Planck's Law. The formula used for calculating the brightness temperature \( T_b \) in radio wavelengths is:\[ T_b = \frac{\lambda^2 B(T)}{2k} \]where:
- \( \lambda \) is the wavelength,
- B(T) is the brightness at temperature T,
- k is the Boltzmann constant.
Consider a star observed at a specific wavelength of 0.5 meters with a measured brightness of 0.4 W/m²/Hertz. To find its brightness temperature:Using the formula:\[ T_b = \frac{(0.5)^2 \, \times \, 0.4}{2 \, \times \, 1.38 \, \text{{x}} \, 10^{-23}} \]The calculations will yield the brightness temperature in Kelvin, however, ensure all values are properly converted.
In atmospheric science, brightness temperature also helps to understand Earth's surface conditions and cloud formations through satellite data. For instance, different ground surfaces show varying brightness temperatures based on their material properties and moisture levels. Instruments on satellites measure this, providing vital data for weather predictions and climate monitoring.
While working with these formulas, always confirm that units are consistent before plugging numbers into your calculations.
Applications of Brightness Temperature Calculation
Brightness temperature is not just theoretical; it has several practical applications, including:
- Astronomy: Helping astronomers understand the energy distribution of stars and galaxies.
- Weather Forecasting: Using satellite data to determine ocean temperatures.
- Radio Telescopes: Used in radio astronomy to map out cosmic microwave background radiation.
Keep in mind, brightness temperature can vary significantly between visible and other electromagnetic spectrum ranges due to the surface emissivity features.
Brightness Temperature Applications in Astrophysics
In the field of astrophysics, understanding the concept of brightness temperature is crucial for analyzing the characteristics of celestial bodies. It provides vital evidence on how these bodies irradiate energy, especially in radio frequencies.
Applications in Observing Celestial Bodies
Brightness temperature has a broad range of applications when it comes to observing different kinds of celestial bodies, including stars, galaxies, and other astronomical phenomena. By measuring radio emissions, astronomers can gather data on:
- Star Composition: Determining the materials present in stars based on their emitted radiation.
- Galaxy Mapping: Utilizing radio telescopes to map out galaxies and their properties.
- Black Hole Studies: Inferring properties of black holes through the radio emissions from accretion disks.
An example in radar astronomy involves measuring the brightness temperature of the planet Jupiter at radio wavelengths. Calculating a brightness temperature of approximately 150 K at these frequencies allows researchers to deduce information about its atmospheric dynamics and composition.
In cosmic microwave background (CMB) studies, brightness temperature is utilized to explore the remnants of radiation left from the Big Bang. By measuring the CMB, which has a brightness temperature of about 2.7 K, scientists can extract information about the universe's early stages, supporting theories about cosmic inflation and large-scale structure formation.
Brightness temperature is often used alongside other measurements, such as spectral lines, to enhance the understanding of complex astronomical systems.
The Role of Brightness Temperature in Radio Astronomy
Radio astronomy relies heavily on the concept of brightness temperature to interpret signals from space. This method provides insights into the thermal and non-thermal processes occurring in space. Understanding its role is pivotal in:
- Non-thermal Emission Identification: Differentiating between thermal and synchrotron emissions in radio sources.
- Cosmic Event Tracking: Monitoring celestial events such as supernovae through radio waves.
- Probing Star Formation: Investigating protostars and star-forming regions.
Keep in mind that the brightness temperature can appear significantly different at varied wavelengths due to changes in emission characteristics across the spectrum.
Uses of Brightness Temperature in Astronomy
In astronomy, the use of brightness temperature is indispensable for evaluating various properties of celestial objects. It makes it possible to translate observed radio waves from space into meaningful information, shedding light on the physical characteristics of these distant phenomena.
Applications for Investigating Celestial Bodies
Brightness temperature aids in the examination of different celestial bodies by providing data on their radio emissions. This information is particularly useful when exploring:
- Planetary Atmospheres: Understanding the temperature distribution in planets like Jupiter.
- Solar Activities: Observing solar flares and sunspots.
- Circumstellar Disks: Assessing young stellar objects and their potential for planet formation.
An example of brightness temperature application can be found in observing the Crab Nebula. At certain radio frequencies, measurements reveal a brightness temperature exceeding 1,000 K, helping astronomers understand its emissions and energetic processes.
Brightness temperature extends beyond visible astronomy into radio observations, allowing for the analysis of cosmic radio sources. With the help of radio telescopes, researchers can detect features such as active galactic nuclei (AGN) and quasars, which possess exceptionally high brightness temperatures indicating intricate energetic processes. The study of such remarkable objects opens avenues to understanding extreme environments far beyond our solar system.
Brightness temperature can reveal information about non-thermal processes, such as synchrotron radiation, by indicating unusually high temperatures.
Role in Monitoring Cosmic Radiation
Another significant use of brightness temperature is in examining cosmic microwave background radiation. This radiation, left over from the early universe, presents a bright uniform glow detected at around 2.7 K. Brightness temperature calculations here enable:
- Early Universe Studies: Understanding the distribution of matter post-Big Bang.
- Cosmological Changes: Charting small fluctuations in brightness temperature across the sky.
- Cosmic Structure Formation: Inferring the growth and formation of cosmic structures over billions of years.
When interpreting cosmic microwave background data, remember that even small variations in brightness temperature can signal significant cosmic events.
brightness temperature - Key takeaways
- Brightness Temperature Definition: The temperature at which a black body emits the same amount of radiation as an object at a specific frequency.
- Brightness Temperature Calculations: Calculated using Planck's Law, particularly at radio wavelengths with the formula: \[ T_b = \frac{\lambda^2 B(T)}{2k} \]
- Differences in Brightness and Physical Temperature: Brightness temperature often exceeds actual physical temperature due to imperfect radiation emission by real objects.
- Applications in Astrophysics: Used to infer properties of stars, galaxies, and black holes by analyzing radio emissions.
- Uses in Astronomy: Invaluable for studying cosmic microwave background radiation, providing insights into early universe conditions around 2.7 K.
- Role of Radio Telescopes: Brightness temperature assists in mapping cosmic phenomena, such as cosmic microwave background and synchrotron emissions, revealing the structure and evolution of astronomical systems.
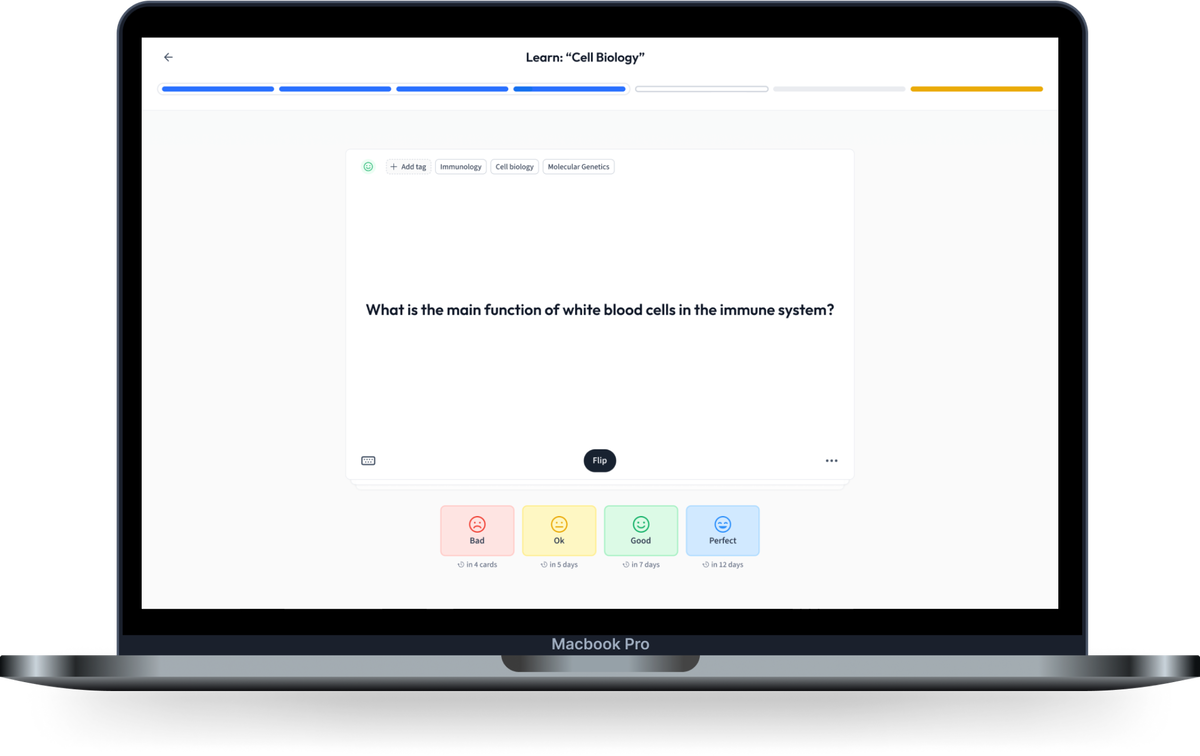
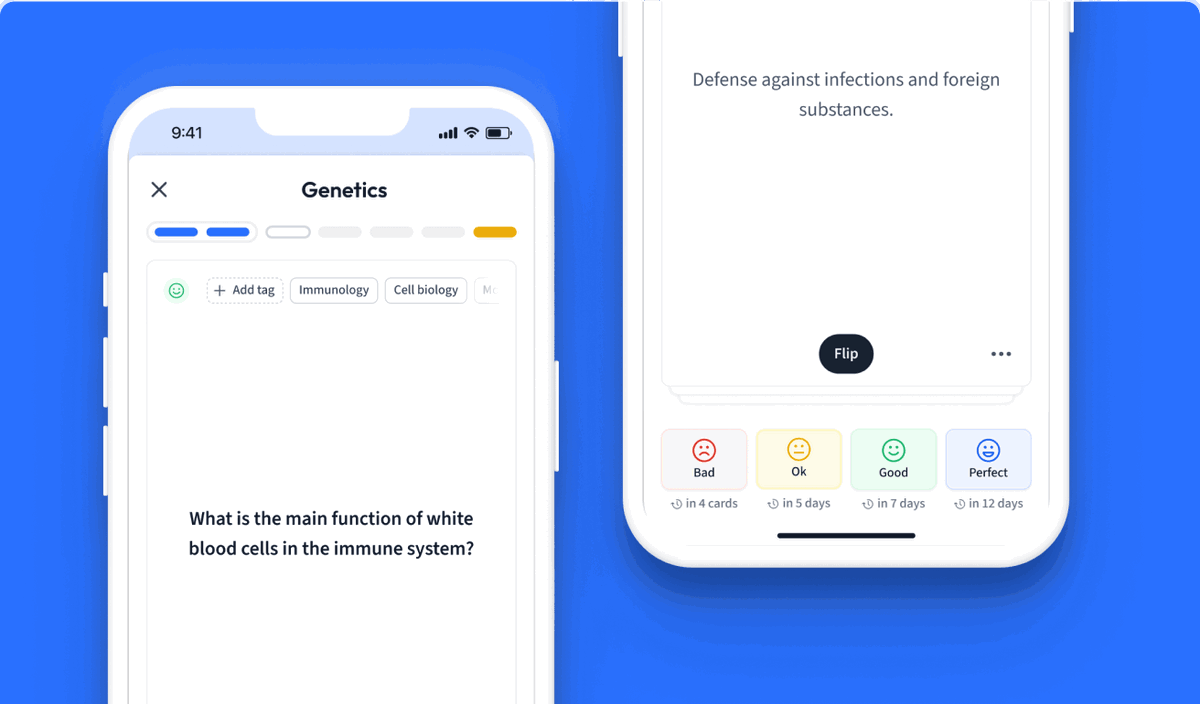
Learn with 12 brightness temperature flashcards in the free StudySmarter app
Already have an account? Log in
Frequently Asked Questions about brightness temperature
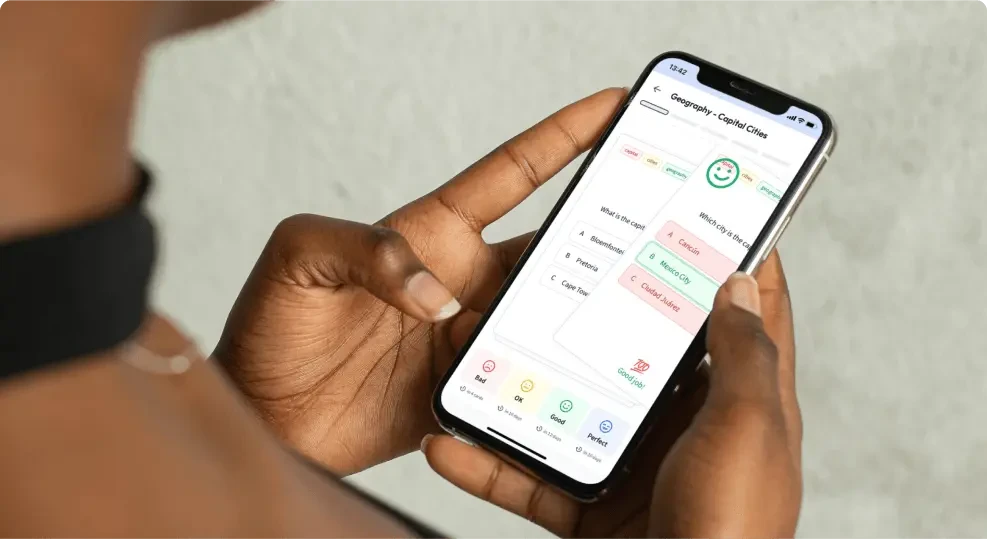
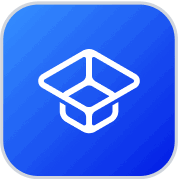
About StudySmarter
StudySmarter is a globally recognized educational technology company, offering a holistic learning platform designed for students of all ages and educational levels. Our platform provides learning support for a wide range of subjects, including STEM, Social Sciences, and Languages and also helps students to successfully master various tests and exams worldwide, such as GCSE, A Level, SAT, ACT, Abitur, and more. We offer an extensive library of learning materials, including interactive flashcards, comprehensive textbook solutions, and detailed explanations. The cutting-edge technology and tools we provide help students create their own learning materials. StudySmarter’s content is not only expert-verified but also regularly updated to ensure accuracy and relevance.
Learn more