Jump to a key chapter
What is Gravitational Lensing?
Understanding gravitational lensing opens a window into the complexities of space and time. This captivating phenomenon not only confirms Einstein’s theory of General Relativity but also helps astronomers study the universe in unique ways.
Gravitational Lensing Definition
Gravitational lensing is the bending of light around massive objects, such as galaxies or black holes, resulting in distorted or multiple images of distant objects.
An effect of the gravitational field on light, it aligns perfectly with predictions made by Einstein’s theory of General Relativity. This bending occurs because mass curves the fabric of space-time, altering the path of light traveling through it.
Gravitational lensing is divided into three primary types:
- Strong Lensing: Evident when multiple distinct images of a background source are visible.
- Weak Lensing: Produces small distortions in shapes of galaxies that require statistical analysis.
- Microlensing: Causes temporary brightness increases when a massive object passes between an observer and a source.
Consider observing a distant galaxy through a telescope. If a massive object, like another galaxy or a black hole, is positioned between you and the distant galaxy, the light will bend, and you may see multiple images, arcs, or a halo around the intervening object due to gravitational lensing.
The first strong evidence of gravitational lensing was observed during a solar eclipse in 1919, supporting Einstein's predictions.
How Gravitational Lensing Works
Gravitational lensing works because light travels along the curved paths dictated by space-time. As light from a distant source, say a star or quasar, passes near a massive object, like a galaxy or black hole, the mass warps the space around it. This warping changes the trajectory of the light, allowing it to reach the observer from different directions.
You can think of it like a glass lens bending light to form a focus or image. Similarly, massive objects in space act as lenses, altering the shape and position of observed celestial objects.
Mathematically, the angle of bending, \( \alpha \), is given by:
\[ \alpha = \frac{4GM}{c^2b} \]
where:
- G is the gravitational constant.
- M is the mass of the object causing the lensing.
- c is the speed of light.
- b is the impact parameter, or the perpendicular distance from the light path to the center of mass.
The phenomenon is not only visually intriguing but plays a crucial role in astronomy. Gravitational lensing helps in detecting dark matter—the invisible matter that doesn't emit light but exerts gravitational forces. By analyzing light distortions, astronomers can map the distribution of dark matter in galaxies and clusters. Moreover, gravitational lensing allows scientists to observe some of the most distant galaxies and objects in the universe by magnifying them.
Types of Gravitational Lensing
Gravitational lensing is a phenomenon dependent on massive objects bending light due to their intense gravitational fields. This creates intriguing and varied effects, categorized primarily into different types based on the distortion's scale and nature.
Strong Gravitational Lensing
Strong gravitational lensing occurs when a massive cosmic object, such as a galaxy cluster, lies between an observer and a distant source. The resulting gravitational field is powerful enough to produce significant distortions.
Here, the light from the background is bent around the lens, forming multiple images, arcs, rings (often called Einstein rings), and sometimes intricate patterns.
In strong gravitational lensing, light from a single source is split into multiple separate images, producing features such as Einstein rings, where light forms a circle around the massive lensing object.
An example of strong gravitational lensing is when a distant quasar's light passes by a massive galaxy, resulting in multiple images on Earth. Consider this scenario: the quasar's light bends around the galaxy, creating distinct and visible images separated at varying angles.
Strong lensing is particularly useful for measuring the mass of lenses, including both visible and dark matter components.
Weak and Microlensing
Weak gravitational lensing deals with subtle distortions that are not detectable on a single image basis. Instead, researchers look at large samples of galaxies for statistical analysis to notice how their shapes are systematically stretched. Weak lensing enables the mapping of matter distribution, notably dark matter, across the universe.
In contrast, microlensing focuses on transient brightening events. Here, smaller objects like individual stars act as lenses, temporarily increasing the brightness of a more distant star. The light of the background star bends slightly, and during this short alignment, it causes magnification.
Weak lensing illustrates how galaxies can appear slightly sheared due to nearby mass concentrations. For instance, the cosmic shear, a form of weak lensing, helped in discovering the large-scale structure of the universe. The observed distortions are used to infer the presence of unseen mass.
Microlensing has been instrumental in detecting exoplanets. As a star and its planet can both act as lenses, this method provides information about extrasolar planets otherwise unreachable by traditional detection techniques. Each microlensing event follows specific patterns mathematically defined by:\[ A(t) = \frac{u^2 + 2}{u\text{√}(u^2 + 4)} \] where \( A(t) \) is the magnification at time \( t \), and \( u \) is the angular position of the source relative to the lens.
Black Hole Gravitational Lensing
Black holes, known for their immense gravitational pull, serve as fascinating subjects for gravitational lensing studies. Their ability to bend light to extreme extents results in unique observational phenomena.
Effects of Black Holes on Light
When light from a distant star passes near a black hole, its path is significantly bent due to the intense gravitational field. This results in distorted or even multiple images of the same astronomical object.
Key effects produced by black hole lensing include:
- Einstein Rings: Circular light patterns around the black hole formed when the source, lens, and observer are perfectly aligned.
- Light Magnification: Amplification of light brightness from the background object, allowing astronomers to detect and study faint celestial bodies.
- Photon Sphere: A hypothetical region where gravity is so strong that even photons travel in circular orbits around the black hole.
An example can be seen when a star's light closely passes a black hole, producing a high magnification event. Consider the scenario of a black hole at a closer proximity than usual, causing noticeable bending and scattered light trails extending from the black hole's glare.
These effects are crucial because they aid in locating invisible black holes and exploring objects otherwise too faint to be seen.
The gravitational field of a black hole is so powerful because it warps space-time itself, making traditional paths of light seem curved.
The mathematical framework describing light bending involves the Schwarzschild metric. The bending angle \( \alpha \) in terms of the Schwarzschild radius \( r_s \) and distance \( r \) is
\[ \alpha = \frac{4r_s}{r} \]
where \( r_s = \frac{2GM}{c^2} \), the formula defining the Schwarzschild radius, incorporates:
- G: Gravitational constant.
- M: Mass of the black hole.
- c: Speed of light.
Observations and Examples
Black hole gravitational lensing has been pivotal in astronomical observations. Telescopes equipped to detect such phenomena can capture and study light bent around black holes, leading to discoveries of various kinds.
Several astronomical projects have utilized gravitational lensing to observe distant and faint objects, contributing to the understanding of the universe’s structure.
A famous example is the Galactic Black Hole known as Sagittarius A*, where researchers observe light from stars and gas spiraling close to it, giving insights into its mass and spin.
Moreover, during certain events where stars align behind this black hole, its gravitational influence can serve to focus light strongly—detecting and studying these events provides data on both the black hole's properties and the background stars.
Gravitational lensing is not only useful for studying the black holes but also for testing the limits of general relativity and our understanding of the cosmos.
Observations of black hole lensing are critical in testing general relativity. They also provide clues about the distribution of dark matter in and around galaxies.
For an even deeper understanding, consider how gravitational waves interact with light near a black hole—a speculative arena where advanced theory meets emerging technology in astronomy. As these waves propagate, they can affect the light path, introducing new subtleties to the lensing effect. Such interactions offer potent insights into both the dynamics of spacetime around black holes and the large-scale structure of the universe itself.
Dark Matter and Gravitational Lensing
Gravitational lensing provides vital insights into numerous cosmic phenomena, notably in the quest to understand dark matter. This elusive substance makes up a significant portion of the universe's mass, impacting the gravitational forces observed in galaxy distributions.
Dark Matter Gravitational Lensing
Dark matter doesn't emit or absorb light, making it invisible to traditional observational methods. Yet its gravitational influence can bend light, making gravitational lensing a powerful tool to detect and map it.
Gravitational lensing allows astronomers to study the warping effects unseen matter like dark matter has on light from more distant sources. As a result:
- It helps in estimating the mass distribution of dark matter within galaxy clusters.
- Provides crucial insights into the interaction between visible and dark portions of the universe.
The phenomenon of dark matter gravitational lensing refers to how light's path is altered by mass structures, composed of dark matter, between the source and the observer.
Imagine looking through a distorted window at a bright light bulb. The light appears scattered and misshaped. Similarly, gravitational lensing distorts the image of galaxies behind clusters filled with dark matter. Measurements of these distortions allow astronomers to infer the presence and distribution of dark matter, even if it's invisible.
Although dark matter itself cannot be observed, gravitational lensing effects provide evidence of its presence and characteristics.
Role in Detecting Dark Matter
The role of gravitational lensing in detecting dark matter is indispensable. As light from background galaxies passes through areas filled with dark matter, its path bends, helping to map the dark matter's distribution.
By analyzing these distortions, scientists can:
- Deduce dark matter's presence and influence on light.
- Estimate how gravitational forces due to dark matter shape galaxies and clusters.
- Refine current cosmological models of the universe's structure.
Key measurements of dark matter's gravitational lensing are achieved through observing weak and strong gravitational lensing effects. For instance, the weak lensing effect relies on statistically analyzing slight shape distortions of millions of galaxies. This requires precision and often involves using complex algorithms to untangle minute distortions from observational noise.
Furthermore, the results from lensing studies have expanded our understanding of the Lambda Cold Dark Matter (ΛCDM) model, the most widely accepted cosmological model of the universe. The shapes of galaxy distributions, combined with the data on cosmic microwave background radiation, are helping to provide a more complete understanding of the universe's age, structure, and evolution.
Mathematically, the bending angle of light, attributed to dark matter’s gravitational influence, can be approximated by Einstein’s lens equation:
\[ \theta_D = \theta_S + \frac{D_{LS}}{D_S} \times \theta_G \]
where:
- \( \theta_D \) designates the deflection angle.
- \( \theta_S \) is the source angle.
- \( D_{LS} \) and \( D_S \) represent the angular diameter distances between lens, source, and observer.
- \( \theta_G \) refers to the gravitational potential's impact angle.
gravitational lensing - Key takeaways
- Gravitational Lensing Definition: The bending of light around massive objects, like galaxies or black holes, resulting in distorted or multiple images of distant objects.
- How Gravitational Lensing Works: Light travels along curved paths due to space-time warped by massive objects, altering its trajectory to reach observers from different directions.
- Types of Gravitational Lensing: Includes strong lensing with multiple distinct images, weak lensing with statistical distortions, and microlensing causing temporary brightness changes.
- Strong Gravitational Lensing: Occurs when a massive object like a galaxy cluster creates significant distortions, forming images, arcs, rings (Einstein rings).
- Black Hole Gravitational Lensing: Black holes bend light to produce unique effects such as Einstein rings, light magnification, and the photon sphere.
- Dark Matter Gravitational Lensing: Used to detect and map dark matter by observing light distortions which indicate mass distributions of invisible matter.
Learn faster with the 12 flashcards about gravitational lensing
Sign up for free to gain access to all our flashcards.
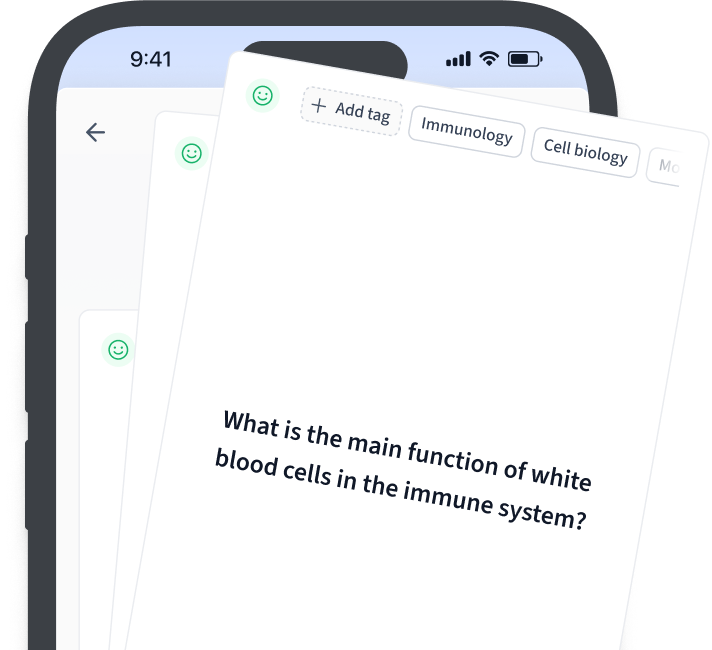
Frequently Asked Questions about gravitational lensing
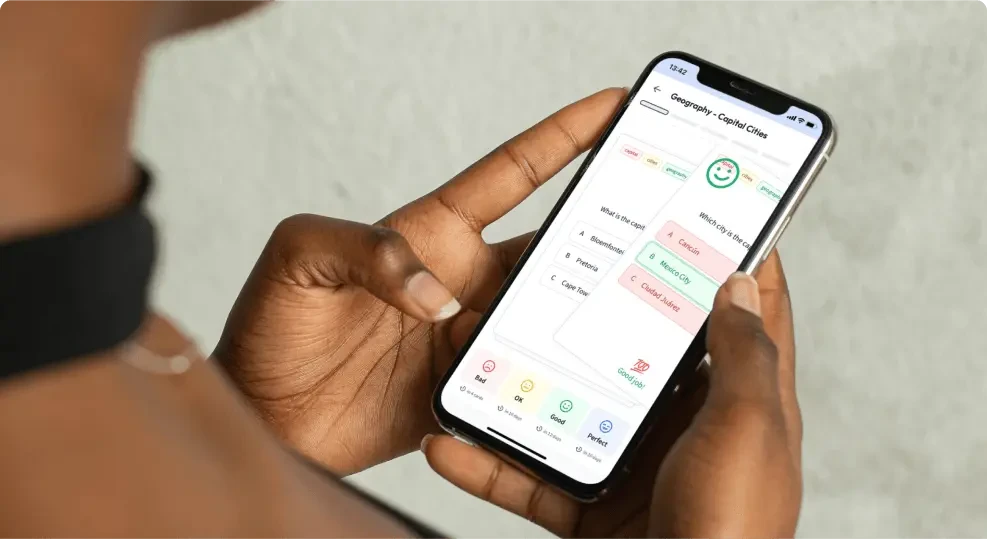
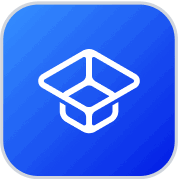
About StudySmarter
StudySmarter is a globally recognized educational technology company, offering a holistic learning platform designed for students of all ages and educational levels. Our platform provides learning support for a wide range of subjects, including STEM, Social Sciences, and Languages and also helps students to successfully master various tests and exams worldwide, such as GCSE, A Level, SAT, ACT, Abitur, and more. We offer an extensive library of learning materials, including interactive flashcards, comprehensive textbook solutions, and detailed explanations. The cutting-edge technology and tools we provide help students create their own learning materials. StudySmarter’s content is not only expert-verified but also regularly updated to ensure accuracy and relevance.
Learn more