Jump to a key chapter
Polarimetry is an important technique in physics that involves the measurement and interpretation of the polarization of light. This method is utilized in many areas, including chemistry, astronomy, and material science.
The principles of polarimetry are based on the alteration of light waves as they pass through various materials. You need to understand the concept of polarized light, which is light whose waves vibrate in a single plane.
Polarimetry experiments often rely on Brewster's angle to achieve optimal polarization by reflection. At this angle, the reflected light is perpendicular to the refracted light. This is mathematically represented as:
\[ \tan(\theta_B) = \frac{n_2}{n_1} \]
where \(n_1\) and \(n_2\) are the refractive indices of the two media involved.
Polarimetry can reveal information about the molecular structure and concentration of substances, exploiting the optical activity of certain materials that rotate the plane of polarization.
You might wonder how polarimetry works. The process generally involves two main components: a light source and a detector. A light source emits unpolarized light which passes through a polarizer, transforming it into polarized light. This light then interacts with the sample under study.
Upon passing through the sample, some materials rotate the plane of the polarized light due to their optical activity, a phenomenon known as optical rotation. The amount of rotation can be measured using a second polarizer, called an analyzer, and is used to derive properties about the sample. Mathematically, the rotation angle \(\alpha\) is expressed by the formula:
\[ \alpha = [\alpha]_T \, c \, l \]
where:
- \([\alpha]_T\) is the specific rotation at temperature \(T\)
- c is the concentration of the solution
- l is the path length through which the light travels
Applications of polarimetry can be extensive, including determining concentrations of molecules and characterizing complex molecular structures.
Diving deeper into polarimetry techniques, you might be interested in exploring ellipsometry, a sophisticated form of polarimetry. It measures the change in polarized light after interaction with a surface. Unlike linear polarimetry, which measures the angle of rotation of the polarization plane, ellipsometry provides two main parameters: the amplitude ratio, \(\tan(\Psi)\), and the phase difference, \(\Delta\).
This technique requires understanding the complex reflection coefficients for parallel (\(r_p\)) and perpendicular (\(r_s\)) polarizations, defined as:
\[ \rho = \frac{r_p}{r_s} = \tan(\Psi) e^{i\Delta} \]
Ellipsometry is highly sensitive to film thickness, refractive index, and surface roughness, making it crucial for characterizing thin films and interfaces in various scientific fields.
Let's look at an overview of the various polarimetry techniques. Each technique is tailored for specific conditions and types of information needed. The most basic setup involves a polarizer and an analyzer. This configuration is straightforward yet effective for many general applications.
Several key techniques include:
- Optical Rotatory Dispersion (ORD): Measures the variation in optical rotation with the wavelength of light.
- Circular Dichroism (CD): Analyzes differential absorption of left and right circularly polarized light, revealing information about the chiral nature of molecules.
- Infrared/Raman Polarimetry: Employs the properties of polarized infrared or Raman scattered light to study chemical compositions and molecular orientations.
Polarimetry's versatility allows it to adapt to different needs within numerous scientific fields like biology, chemistry, and material science.
The process of polarimetry plays a crucial role in a myriad of scientific disciplines. Its ability to study the polarization of light enables new insights into various natural phenomena.
In the realm of astrophysics, polarimetry sheds light on the fascinating and complex universe. It helps determine the intrinsic properties of light coming from celestial bodies such as stars and galaxies, thereby unraveling their mysteries.
Polarized light from astronomical objects can arise from different processes:
- Scattering: Light can become polarized when scattered by dust and gas in the interstellar medium.
- Magnetic Fields: The presence of magnetic fields can align dust grains, leading to polarized starlight.
- Synchrotron Radiation: Charged particles moving at relativistic speeds in magnetic fields emit polarized light, often observed in supernova remnants and active galactic nuclei.
By measuring the degree and angle of polarization, polarimetry provides vital information about:
- The orientation and strength of magnetic fields.
- The composition and distribution of cosmic dust.
- The geometry and physical properties of emitting and scattering regions.
One exciting application is in studying the cosmic microwave background radiation (CMBR), the afterglow of the Big Bang. Polarimetry helps analyze the polarization pattern imprinted by density fluctuations and gravitational waves, providing insights into the early universe.
Cosmic Microwave Background Radiation (CMBR) is the thermal radiation left over from the Big Bang, providing essential clues about the universe's origin and composition.
Astrophysical polarimetry also extends into the study of exoplanets. When starlight passes through an exoplanet's atmosphere, it becomes polarized to varying degrees. Observing the degree of polarization can provide information about the atmosphere's composition, cloud cover, and surface features. This kind of data is invaluable in the search for potentially habitable planets.
Polarimetry's application isn't confined to astrophysics; its potential spans across numerous scientific disciplines. In fields like chemistry and biology, this technique offers a unique window into molecular structures and their interactions.
In chemistry, polarimetry is used to study chiral molecules, which have non-superimposable mirror images. This property is crucial when analyzing substances with optical activity. For example, the rotation angle of polarized light passing through a sugar solution can reveal its concentration, crucial for quality control and research purposes.
Application | Field | Importance |
Determining Sugar Concentration | Chemistry | Quality Control |
Protein Structure Analysis | Biology | Understanding Protein Functions |
Toxicology Testing | Pharmaceuticals | Drug Safety |
In biology, Circular Dichroism (CD) is a form of polarimetry employed to assess the secondary structures of proteins and nucleic acids. The differential absorption of left and right circularly polarized light provides insights into the conformational changes in biomolecules under various conditions, which is pivotal in drug design and development.
Consider a practical example with protein analysis. By employing polarimetry, researchers can determine how a protein folds and changes in structure when exposed to a new environment. This information is critical in understanding how proteins function in biological processes or an immune response.
Did you know? Polarimetry can also be used in meteorology to study weather patterns, particularly by analyzing rain and cloud droplets.
In material sciences, polarimetry aids in the characterization of thin films and surfaces. Techniques like ellipsometry are instrumental in providing detailed information about optical properties and film thickness. This information is essential in the development and optimization of coatings, photovoltaic cells, and nano-layered materials.
Polarimetry, a fundamental technique in physics, plays a vital role in analyzing the properties of light, particularly in terms of its polarization. Delving into its historical roots and contemporary applications reveals its extensive impact in science.
The history of polarimetry dates back to the early 19th century when its principles were first unveiled. The fascination with light and its mysterious properties sparked curiosity among scientists of the era. Among the pioneers were Étienne-Louis Malus, who discovered the polarization of light by reflection, and Auguste Fresnel, who developed wave-based theories of light.
The early studies focused on understanding how light interacts with surfaces and how certain crystals, like calcite, could split a single light beam into two polarized beams. This phenomenon, known as birefringence, became a significant scientific interest, laying the groundwork for further developments in optics.
Historical techniques involved using Brewster's angle to precisely measure polarization effects. Brewster’s angle is defined by the equation:
\[ \tan(\theta_B) = \frac{n_2}{n_1} \]
where \(n_1\) and \(n_2\) are the refractive indices of the two interfacing media.
An interesting historical example of polarimetry's application was in the sugar industry. By utilizing polarimeters, scientists could accurately measure the concentration of sugar solutions through the specific rotation of light, enhancing quality control in manufacturing processes.
Exploring deeper into historical perspectives, the development of polarimetry was greatly influenced by the study of natural optical activity found in certain organic compounds. In substances like turpentine, the rotation of polarized light provided deep insights into the molecular structures and compositions. The research into optical activity significantly advanced the understanding of stereochemistry, chiral molecules, and even paved the way for modern pharmaceutical applications.
The field of polarimetry has significantly advanced with modern technological developments. Contemporary polarimetry extends beyond the visible spectrum, exploring the infrared and ultraviolet ranges with high precision.
Innovations have led to the creation of sophisticated polarimetric tools, such as automatic polarimeters that offer enhanced sensitivity and accuracy, crucial for research and industrial applications. These instruments employ computer algorithms to analyze polarization data, providing precise measurements of optical rotation and ellipticity.
In recent years, developments have focused on enhancing polarimetry's sensitivity to nanoscale measurements, essential in exploring the properties of nanomaterials and quantum dots. Using advanced techniques like scanning near-field optical microscopy (SNOM), researchers can achieve near-optical resolution at the nanoscale, crucial for material characterization and electronic applications.
Did you know? 3D cinema relies on polarimetry principles. By using polarized glasses, viewers can experience images with depth, creating a three-dimensional effect.
Nanoscale Polarimetry: is a modern advancement in studying materials at the billionth of a meter scale, allowing precise exploration of optical properties in nanotechnology and materials science.
Modern polarimetry also finds applications in environmental science, particularly in atmospheric studies. By measuring the polarized light scattered by atmospheric particles, scientists gain crucial information about pollution levels and atmospheric conditions.
In the quest for renewable energy, polarimetry is used to study the efficiency and properties of photovoltaic materials. It provides insights into the alignment and interaction of molecules within solar cells, aiding in the optimization of energy conversion processes.
The intersection of polarimetry with biomedical imaging signifies another exciting advancement. Techniques like polarimetric OCT (Optical Coherence Tomography) are used to enhance tissue imaging, providing detailed information about tissue structure and composition, enhancing diagnostic capabilities in the medical field.
Polarimetry is a key technique in the field of physics used to measure the polarization of light. This involves analyzing how light interacts with various substances, revealing information about their structure and properties.
To perform polarimetry, specific tools and instruments are essential for accurate measurements of light properties. These instruments range from basic components to advanced technological devices, each serving a unique purpose in the polarimetric process.
Several essential instruments include:
- Polarizers: Devices that filter light waves, allowing only those with certain planes of vibration to pass through. Common types include linear and circular polarizers.
- Analyzers: Used to analyze the polarized light after it interacts with a sample, helping to measure changes in orientation.
- Photoelastic Modulators: These are used to modulate the polarization state of light, particularly beneficial in dynamic measurements.
- Polarimeters: Instruments designed to measure the angle of rotation caused by a substance on passing polarized light. Automatic digital polarimeters are popular due to their precision and ease of use.
In understanding the functioning of these devices, a basic understanding of the optical rotation formula is crucial:
\[ \alpha = [\alpha]_T \, c \, l \]
where:
- \([\alpha]_T\) is the specific rotation.
- c is the concentration of the solution.
- l is the length of the path through which light travels.
Consider using a polarimeter to determine the concentration of a sugar solution. By measuring the optical rotation of polarized light passing through the solution, the concentration can be accurately calculated, proving invaluable in quality control processes in industries such as food and beverages.
Automatic polarimeters with digital displays simplify the measurement process, reducing human error and increasing efficiency in laboratories.
While polarimetry is an insightful technique, it comes with its set of challenges. Addressing these challenges is crucial for enhancing the accuracy and reliability of polarimetric measurements.
Some common challenges in polarimetry analysis include:
- Sample Impurities: Impurities in samples can result in inaccurate measurements by influencing the behavior of polarized light.
- Temperature Variations: Changes in temperature can affect the refractive indices of substances, altering polarization measurements.
- Calibration Issues: Calibration errors in polarimetric instruments may lead to significant measurement deviations.
- Light Scattering: Scattering by particles within a sample can complicate the interpretation of polarization data.
Advanced computational models and improved instrument design continue to address these challenges, enhancing the precision and application of polarimetry in scientific research.
In overcoming these challenges, researchers are exploring adaptive polarimetry techniques that automatically adjust to varying conditions. Innovations include the development of ellipsometry and circular dichroism, which offer greater sensitivity and flexibility in measurements. Ellipsometry, for instance, measures the change in polarization upon reflection or transmission from a material, providing detailed insights into complex refractive indices and layer thicknesses in thin films. The equation for elliptic reflection coefficients is:
\[ \rho = \frac{r_p}{r_s} = \tan(\Psi) e^{i\Delta} \]
where \(r_p\) and \(r_s\) represent the reflection coefficients for parallel and perpendicular polarizations, respectively, with \(\Psi\) and \(\Delta\) symbolizing the magnitude and phase differences.
polarimetry - Key takeaways
- Polarimetry: A technique in physics used for measuring and interpreting the polarization of light across various fields such as chemistry, astronomy, and material science.
- Principles of Polarimetry: Based on the alteration of light waves as they pass through different materials, using concepts like polarized light and optical rotation.
- Brewster's Angle: Utilized in polarimetry experiments to achieve optimal polarization, mathematically defined by the tangent of the angle equating the ratio of refractive indices of two media.
- How Polarimetry Works: Involves a light source, polarizer, and analyzer to measure optical rotation in samples by rotating the plane of polarized light.
- Applications of Polarimetry: Encompass determining molecular concentrations, characterizing molecular structures, and studying atmospheric and astrophysical phenomena.
- Polarimetry Techniques: Include Optical Rotatory Dispersion, Circular Dichroism, and Ellipsometry, each providing unique insights through different approaches.
Learn faster with the 12 flashcards about polarimetry
Sign up for free to gain access to all our flashcards.
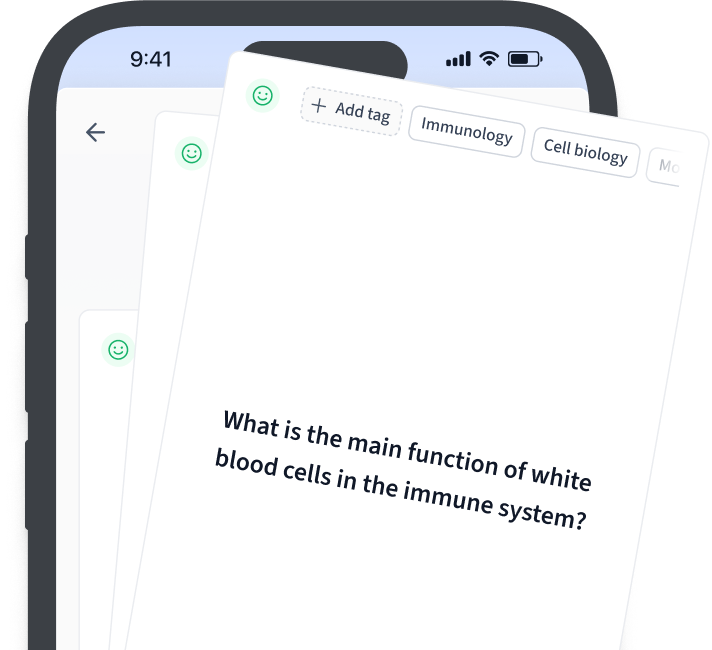
Frequently Asked Questions about polarimetry
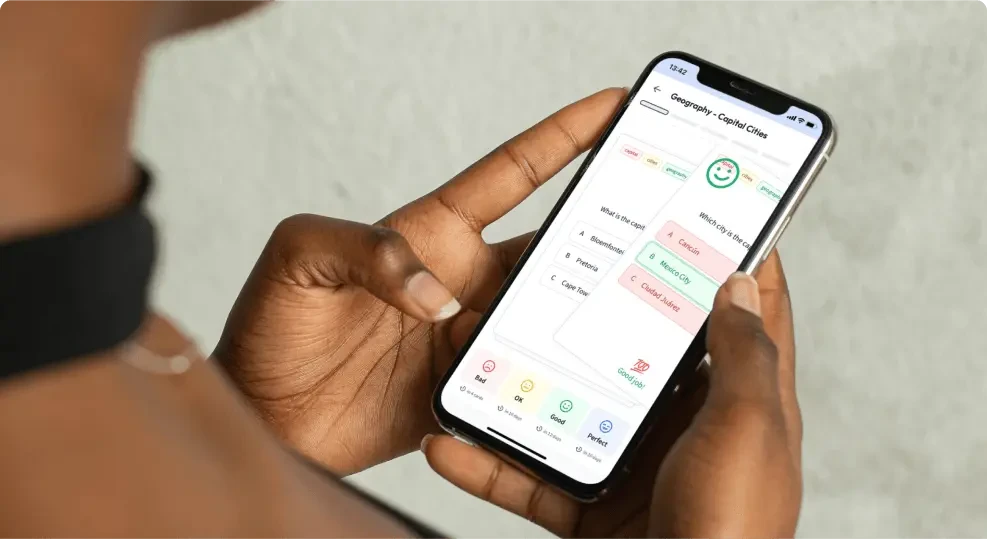
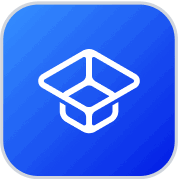
About StudySmarter
StudySmarter is a globally recognized educational technology company, offering a holistic learning platform designed for students of all ages and educational levels. Our platform provides learning support for a wide range of subjects, including STEM, Social Sciences, and Languages and also helps students to successfully master various tests and exams worldwide, such as GCSE, A Level, SAT, ACT, Abitur, and more. We offer an extensive library of learning materials, including interactive flashcards, comprehensive textbook solutions, and detailed explanations. The cutting-edge technology and tools we provide help students create their own learning materials. StudySmarter’s content is not only expert-verified but also regularly updated to ensure accuracy and relevance.
Learn more