Jump to a key chapter
Fundamentals of Radio Astronomy
Radio astronomy is a subfield of astronomy that studies celestial objects by detecting the radio waves they emit. Understanding these fundamentals helps you appreciate how astronomers gather data about the universe beyond the visible spectrum.
Basics of Radio Astronomy Techniques
Radio astronomy techniques are specialized methods used to measure and analyze radio waves from celestial objects. Some primary techniques include:
- Interferometry: Utilizes multiple telescopes to enhance resolution by correlating the signals received at different locations.
- Radio Mapping: Maps the distribution of radio emissions from objects, aiding in understanding their structure.
- Polarimetry: Observes the polarization of radio waves, providing information about magnetic fields.
- Spectroscopy: Analyzes radio frequency spectra to identify chemical compositions and other properties of astronomical objects.
Example of Interferometry: Consider two radio antennas spaced kilometers apart. These antennas collect data on the same source. The combined data improves the image's resolution as if it were captured by a larger antenna opening.
Deep Dive into Interferometry: Interferometry's ability to significantly enhance resolution is due to its reliance on a technique knownas the Very Long Baseline Interferometry (VLBI). By joining telescopes over vast distances, VLBI achieves angular resolution far surpassing single radio antennas,enabling the study of distant celestial objects with unprecedented detail. The formula for resolving power in interferometry can be expressed as:\[\theta = \frac{\lambda}{B}\]where \(\theta\) is the angular resolution, \(\lambda\) is the wavelength of observation, and \(B\) is the baseline length between antennas.
Importance of Radio Astronomy Imaging Techniques
Radio astronomy imaging techniques are essential for visualizing and interpreting the radio data from astronomical sources. These techniques help uncover the universe's hidden facets and provide valuable insights into astronomical phenomena.
Deep Dive into Radio Imaging: Radio imaging transforms data into functional images, illustrating an object's radio emission distribution. Techniquessuch as Fourier Transform and CLEAN algorithm are employed to transform raw data. Fourier Transform rearranges data into identifiablepatterns, while the CLEAN algorithm refines these images by identifying and subtracting artifacts. This combination produces images with greater clarityand fewer distortions, critical for studying complex astronomical sources like galaxies and supernova remnants.
Remember, the resolution of radio images depends on the size and sensitivity of the radio telescope. The larger and more sensitive, the clearer the image.
Radio Interferometry Techniques
Radio interferometry is a powerful technique in radio astronomy that significantly enhances the resolution of astronomical images. By using multiple radio telescopes spread across large distances, this method allows astronomers to observe celestial objects with great precision.The study of radio waves emitted by stars, galaxies, and other astronomical objects requires sophisticated technology and understanding. Interferometry enables observations that a single telescope cannot achieve.
Introduction to Radio Interferometry
The basic principle of radio interferometry involves combining signals from several telescopes that are physically distanced from each other. These signals are then correlated to mimic a single large aperture.The primary advantage of this method is the improvement in angular resolution, which is defined by the formula:\[\theta = \frac{\lambda}{B}\]where \(\theta\) is the angular resolution, \(\lambda\) is the wavelength, and \(B\) is the distance or baseline between the telescopes.
Definition: Angular resolution measures the smallest detail that a telescope can distinguish. Smaller values of \(\theta\) indicate better resolutions.
Example: Suppose two telescopes are situated 1000 meters apart, observing a radio wave with a wavelength of 1 meter. The angular resolution can be calculated as:\[\theta = \frac{1}{1000} = 0.001\] or 1 milliradian.
Large baseline arrays, such as the Very Long Baseline Array (VLBA), use multiple locations worldwide to achieve unprecedented angular resolutions.
Applications of Radio Interferometry Techniques
Radio interferometry offers several applications in radio astronomy, providing critical insights into various cosmic phenomena. These applications include:
- Mapping Pulsars: Provides data on the precise location and behavior of neutron stars. Understanding these objects helps in studies of extreme physics and gravitational waves.
- Studying Quasars: Offers valuable information about distant and active galactic nuclei. Interferometry allows precise measurements of their emitted radio waves.
- Analyzing Cosmic Microwave Background (CMB): Uses large arrays to map fluctuations in the CMB, giving insights into the early universe's conditions.
Deep Dive: Interferometry's application extends to the observation of exoplanets by studying the slight wobbles or emissions that suggest a planet's presence.It plays a vital role in answering questions about planet formation and habitability beyond our solar system.The advancement of techniques such as sparse aperture masking interferometry enables greater precision, especially beneficial in the study of closely orbiting binary stars and exoplanet discovery.
Single-Dish Radio Astronomy Techniques and Applications
Single-dish radio astronomy involves using one large telescope, often parabolic in shape, to collect and focus radio waves from celestial objects. Despite the advent of more intricate techniques like interferometry, single-dish radio astronomy remains pivotal for many observations. The simplicity and versatility of this method are key to its numerous scientific applications.
Understanding Single-Dish Radio Astronomy
Single-dish radio telescopes work by gathering radio signals through a large antenna, directing them to a receiver for amplification and analysis. These telescopes are ideal for certain tasks due to:
- Sensitivity: Suitable for observing faint radio emissions from vast regions of space.
- Wide-field of view: Capable of scanning large areas of the sky efficiently.
- Rapid Surveying: Enables quick identification of transient radio sources.
Effective Area \(A_e\): The area of the telescope that successfully captures incoming radio signals, affecting its sensitivity and efficiency of observation.
Example of Sensitivity: If a radio telescope has a diameter of 100 meters and observes wavelengths of 21 cm:\[A_e = \frac{\text{π} \times (50)^2}{(0.21)^2} \approx 14,000 \text{ m}^2\]This calculation illustrates the telescope's effective area, highlighting its ability to detect weak signals from distant sources.
Larger telescopes typically gather more radio emissions, enhancing sensitivity but requiring more detailed structural design for optimal performance.
Practical Applications of Single-Dish Techniques
Single-dish techniques are foundational in radio astronomy, supporting diverse investigations, such as:
- Monitoring Variable Stars: Observing fluctuations in radio emissions that indicate changes in stellar activity.
- Galaxy Surveys: Conducting extensive surveys to catalog radio emissions from galaxies, aiding in the understanding of galactic structure and evolution.
- Study of Cosmic Magnetism: Analyzing polarization and rotation measures of radio waves to infer the existence and properties of cosmic magnetic fields.
Deep Dive into Galaxy Surveys: One of the major applications of single-dish telescopes is in galaxy surveys. These are essential for piecing together the large-scale structure of the universe.With capabilities to scan vast sky areas uniformly, single-dish observations allow astronomers to map the distribution of hydrogen gas, a primary component in galaxy formation.The survey's success relies on measuring the 21-cm hydrogen line, as the specific radio emission serves as a universal tracer to study the formation and evolution of galaxies.Using the doppler shift formula: \[v = \frac{(u - u_0)}{u_0}c \] where \(u\) is the observed frequency, \(u_0\) is the rest frequency, and \(c\) is the speed of light, astronomers can determine relative velocities of galaxies, crucial for understanding cosmic expansion.
Techniques in Radio Astronomical Data Analysis
Radio astronomy relies heavily on sophisticated data analysis techniques to interpret the extensive data collected from radio telescopes. These analyses enable you to understand the physical characteristics and processes occurring in celestial objects.
Data Analysis in Radio Astronomy
In radio astronomy, data analysis involves numerous steps to ensure the integrity and accuracy of the observations. Key steps include:
- Data Collection: Gathering raw data from radio telescopes, capturing frequencies emitted by astronomical bodies.
- Data Reduction: Processing data to remove noise and interference. This includes correcting for atmospheric effects and instrument errors.
- Data Calibration: Adjusting the data using known reference sources for accurate analysis.
- Data Imaging: Creating visual representations from radio signals to study the structure and behavior of astronomical objects.
Deep Dive into Data Calibration: Calibration is critical for maintaining data accuracy, accounting for gain variations and other system imperfections. Consider the calibration equation:\[S_{obs} = S_{true} \times G + N\]where \(S_{obs}\) is the observed signal, \(S_{true}\) is the true signal, \(G\) represents gain, and \(N\) is the noise. By adjusting \(G\) and \(N\), you can extract the accurate representation of \(S_{true}\). Advanced methods like self-calibration further refine imaging by iterating through adjusting \(G\) and removing \(N\) based on the data itself.
To enhance data quality, always ensure consistent use of reference sources across calibration sessions.
Exploring Radio Spectrometry Methods
Radio spectrometry provides insights into the composition and properties of celestial objects by examining the spectrum of radio emissions.Key methods within radio spectrometry include:
- Line Spectroscopy: Identifies molecular and atomic lines, revealing the presence of specific elements like hydrogen.
- Spectral Imaging: Constructs a 3D view of astronomical structures by combining spatial and spectral data.
- Continuum Spectroscopy: Analyzes wide frequency ranges to understand thermal emissions and synchrotron radiation.
Example of Line Spectroscopy: Detecting the presence of hydrogen in the interstellar medium involves measuring the 21-cm line.This line is used to calculate the velocity of structures moving along our line of sight using the Doppler shift equation: \[ \Delta u = u_0 \times \frac{v}{c}\]where \(\Delta u\) is the change in frequency, \(u_0\) is the rest frequency, \(v\) is the velocity, and \(c\) is the speed of light.
Deep Dive into Spectral Imaging: This method transforms data into a form where each pixel holds spectral information, akin to a data cube.By slicing this cube along different spectral lines, you can gain insights into various components of the observed astronomical object.For example, studying a galaxy across multiple slices might reveal:
- Gas distribution in different velocity ranges
- Dynamics of rotating star-forming regions
- Effect of magnetic fields on dust particles
radio astronomy techniques - Key takeaways
- Radio Astronomy Techniques: Utilizes specialized methods like interferometry, radio mapping, polarimetry, and spectroscopy to measure and analyze radio waves from celestial objects.
- Radio Interferometry: Enhances image resolution by combining signals from multiple telescopes, using the formula for angular resolution: \(\theta = \frac{\lambda}{B}\) where \(\theta\) is angular resolution, \(\lambda\) is wavelength, and \(B\) is baseline.
- Single-Dish Radio Astronomy: Involves using one large telescope to collect radio signals with applications in sensitivity, wide-field surveying, and galaxy surveys.
- Radio Spectrometry Methods: Includes line spectroscopy, spectral imaging, and continuum spectroscopy to understand the composition and properties of celestial objects.
- Data Analysis in Radio Astronomy: Processes include data collection, reduction, calibration, and imaging to ensure accuracy and extract meaningful insights.
- Radio Astronomy Imaging Techniques: Techniques like Fourier Transform and CLEAN algorithm transform data into detailed images for studying complex sources like galaxies.
Learn faster with the 12 flashcards about radio astronomy techniques
Sign up for free to gain access to all our flashcards.
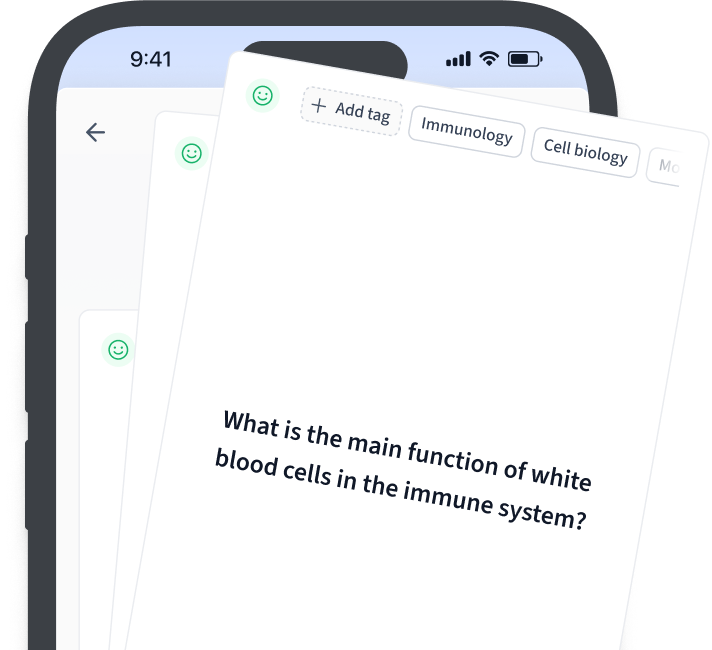
Frequently Asked Questions about radio astronomy techniques
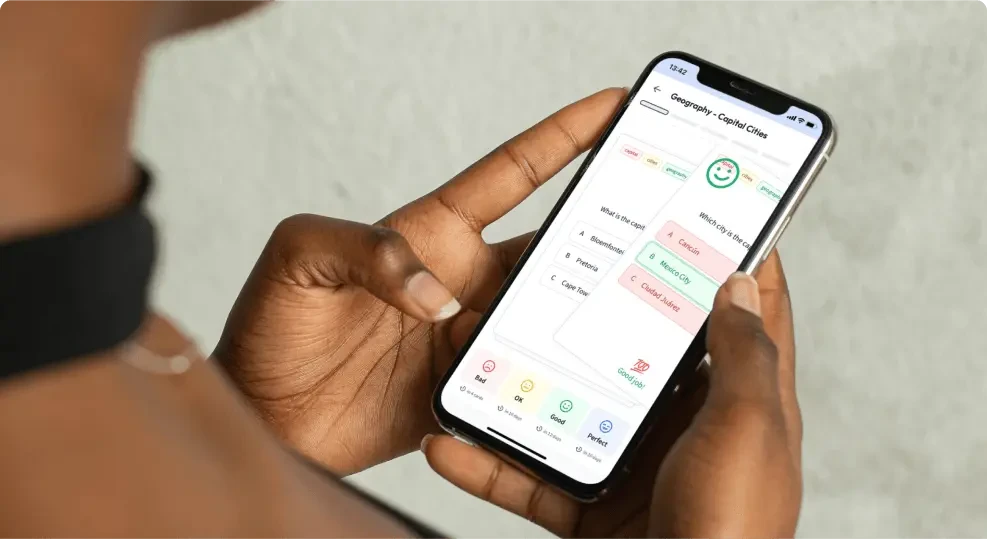
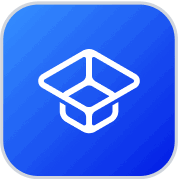
About StudySmarter
StudySmarter is a globally recognized educational technology company, offering a holistic learning platform designed for students of all ages and educational levels. Our platform provides learning support for a wide range of subjects, including STEM, Social Sciences, and Languages and also helps students to successfully master various tests and exams worldwide, such as GCSE, A Level, SAT, ACT, Abitur, and more. We offer an extensive library of learning materials, including interactive flashcards, comprehensive textbook solutions, and detailed explanations. The cutting-edge technology and tools we provide help students create their own learning materials. StudySmarter’s content is not only expert-verified but also regularly updated to ensure accuracy and relevance.
Learn more