Jump to a key chapter
Definition of Synthetic Photometry
Synthetic Photometry is a technique used in astronomy to simulate the photometric properties of celestial objects. It employs computational models to calculate the expected luminosity of objects based on theoretical models and spectral data.The objective of synthetic photometry is to understand and interpret astronomical observations by comparing synthetic spectra with actual observations, enabling astronomers to derive physical properties such as temperature and chemical composition.
Methodology of Synthetic Photometry
The process of synthetic photometry involves several steps that combine theory, data analysis, and computation. Here’s a general outline of how it works:
- Data Collection: Obtain spectral data of a star or astronomical object either from observations or theoretical models.
- Modeling: Use theoretical models to simulate the expected output spectrum of the object. This involves generating models of the star's atmosphere and predicting its spectral distribution.
- Filter Simulation: Apply photometric filters to these spectra to predict the brightness of the object in various passbands.
- Comparison: Compare these synthetically generated photometric data with observed results to check the accuracy and refine models.
Imagine you have a star with a known temperature and chemical composition. Using synthetic photometry, you can model how this star would appear in different photometric filters used by telescopes. If your model predicts the star would be very bright in the ultraviolet band, but observations show it is dimmer, it might suggest something interesting, like the presence of interstellar dust.
Synthetic photometry can be particularly useful in large-scale surveys, where telescopes collect data over a broad range of frequencies.
For a deeper understanding, you could explore how synthetic photometry applies to calibrating photometric zero-points. Photometric zero-points are crucial because they anchor the observed brightness to a physical scale. Consider an ultraviolet filter with response function \(R(\lambda)\). The observed flux \(F_{\text{obs}}\) can be compared against a modeled spectrum \(F_{\text{model}}(\lambda)\) using:\[ m = -2.5 \log \left( \frac{\int R(\lambda) F_{\text{obs}}(\lambda) d\lambda}{\int R(\lambda) F_{\text{model}}(\lambda) d\lambda} \right) + ZP \]where \(ZP\) is the zero-point of the photometric system. This calibration ensures that synthetic data aligns precisely with observational results. Exploring this facet reveals how synthetic photometry serves as a bridge between theoretical predictions and empirical data.
Meaning of Synthetic Photometry
Synthetic Photometry is the astronomical technique used to simulate the photometric properties of celestial objects using computational models. By comparing synthetic results with real observations, astronomers can deduce properties like temperature and chemical compositions.The methodology involves incorporating theoretical spectra, applying photometric filters, and deriving synthetic magnitudes that mirror observational data.
Synthetic Photometry: A computational approach to simulate and analyze the photometric properties of space phenomena by utilizing synthetic spectral data and theoretical modeling.
The process of synthetic photometry typically includes several key steps:
- Data Collection: Spectral data gathering from astronomical sources or models.
- Computational Modeling: Developing and validating models of the object's spectrum using theoretical calculations.
- Filter Application: Simulating how photometric filters transform the spectrum into measurable fluxes across distinct bands.
- Analysis: Comparing synthetic data to observational results aids astronomers in verifying hypotheses and refining models.
Suppose you encounter a star whose luminosity distribution you want to study. Using synthetic photometry, its spectrum can be modeled corresponding to various filter bands. If the synthetic data indicates more infrared brightness compared to rendered actual observation, it might suggest interstellar medium effects or intrinsic stellar properties altering its emission.
Bringing theoretical predictions close to observational data is where synthetic photometry showcases its importance. The comparison leverages the integral:\[ F_{\text{filter}} = \frac{\int R(\lambda) F(\lambda) d\lambda}{\int R(\lambda) d\lambda} \]Here, \( F(\lambda) \) denotes the spectrum, and \( R(\lambda) \) signifies the filter's response function.
Synthetic photometry also contributes significantly to the calibration of telescopic instruments, ensuring data quality across varying conditions.
Delving deeper into its applications, synthetic photometry is integral to developing stellar atmosphere models. It helps refine stellar classifications based on temperature, gravity, and composition by fitting spectral models to observable magnitudes. Consider the Balmer series absorption lines. By analyzing these lines in a star's spectrum, synthetic photometry aids in determining the star's atmospheric parameters like effective temperature \( T_{\text{eff}} \) and surface gravity \( \log g \). Theoretical models simulate these parameters, providing insights into a star's evolutionary state and chemical makeup. Understanding how these lines behave under different conditions enables astronomers to classify stars more accurately.
Synthetic Photometry Techniques
Synthetic photometry techniques play a vital role in understanding the physical properties of celestial objects. By using computational tools to simulate and analyze spectral data, these techniques support astronomers in drawing connections between theoretical predictions and observational data. This involves a sequence of well-defined steps enabling accurate analysis and modeling of astrophysical phenomena.
Common Synthetic Photometry Techniques
Common synthetic photometry techniques used in astronomy assist in the basic understanding of photometric data and its applications:
- **Filter Simulation:** Applying known filter properties to a spectrum to derive expected photometric magnitudes. This typically employs the response function \(R(\lambda)\).
- **Spectrum Synthesis:** Using physical models to create theoretical spectra that match observed spectra by adjusting parameters like metallicity or surface gravity.
- **Photometric Calibration:** Ensuring photometric measurements are consistent across different instruments or observing conditions by applying synthetic magnitudes.
Technique | Description |
Filter Simulation | Uses photometric filters to derive magnitudes from theoretical spectra. |
Spectrum Synthesis | Adjusts model parameters to fit observed spectra. |
Photometric Calibration | Aligns observational data with theoretical predictions. |
Consider an astronomical survey capturing data in the visible light spectrum. By simulating the spectrum of stars in this survey using filters like Johnson B and V bands, synthetic photometry helps determine the expected luminosity and compare these against actual measurements. For a star with known apparent magnitude in one filter, the conversion to another can be determined using:\[ m_{B} - m_{V} = -2.5 \log \left( \frac{F_B}{F_V} \right) \]where \(F_B\) and \(F_V\) are fluxes in the B and V bands, respectively.
Inconsistencies between synthetic and observed magnitudes can hint at unknown physical processes or data processing errors.
Advanced Synthetic Photometry Techniques
Advanced techniques in synthetic photometry delve deeper into fine-tuning models and interpreting complex data:
- **Radiative Transfer Models:** These simulations include not only basic spectral synthesis but also radiative processes within the stellar atmosphere, allowing for more accurate spectrum modeling.
- **Multi-spectral Analysis:** By analyzing data across a wide range of wavelengths—from X-ray to radio—multi-spectral techniques offer comprehensive views of stellar properties.
- **Stellar Evolution Codes:** Integrating synthetic photometry with stellar evolution codes helps predict the photometric properties of stars through various stages of evolution.
Exploring the application of thermal inversion layers, advanced synthetic photometry delves into radiative transfer models to understand how layers within a star's atmosphere reverse expected thermal profiles, influencing observed spectra. For example, the synthetic spectrum of a star calculated including thermal inversion might feature prominent unusual emission lines due to localized increases in temperature. These anomalies can provide insights into dynamic processes like magnetic activity or accretion phenomena in celestial bodies.
Applications of Synthetic Photometry in Physics
The diverse applications of synthetic photometry in physics make it a powerful tool for analyzing astronomical phenomena. By integrating theoretical models with observational data, synthetic photometry allows for detailed explorations of the universe.
Astrophysical Research and Synthetic Photometry
Astrophysical research heavily relies on synthetic photometry to glean insights into the universe's complexities. By simulating stellar and galactic spectra, researchers can:
- Determine physical characteristics, such as temperature and composition, of distant stars.
- Examine the structure and dynamics of galaxies.
- Analyze the effects of dust and other interstellar materials on light absorption and scattering.
Consider modeling a star cluster using synthetic photometry. By simulating each star’s spectrum under different filters, researchers could identify variations in brightness which may suggest differences in age or chemical composition. Photometers might report magnitudes via:\[ m = -2.5 \log \left( \frac{F}{F_0} \right) \]Here, \( F \) represents the observed flux, and \( F_0 \) is a reference flux.
Expanding further, synthetic photometry facilitates the exploration of stellar population synthesis models, which work to simulate an entire galaxy's composite light. By altering variables like star formation rate and initial mass function in models, astronomers deduce the characteristics of galaxies at various stages of evolution. This data becomes crucial in addressing unresolved questions about galaxy formation and development in the universe.
Synthetic Photometry in Stellar Studies
In stellar studies, synthetic photometry is indispensable for understanding the life cycles of stars. By comparing synthetic spectra against observational spectra, you can:
- Track changes in brightness over time to pinpoint evolutionary stages.
- Study binary star systems and detect companion stars by analyzing combined light.
- Identify unique features, such as emission lines, indicative of stellar phenomena like accretion disks or nebulosity.
Synthetic photometry aids in revealing anomalies attributed to stellar surface activities, like star spots or flares, by matching variability patterns.
Role of Synthetic Photometry in Cosmology
In the field of cosmology, synthetic photometry assists in understanding the universe's large-scale structure and evolution. Through these applications, it:
- Supports the mapping of cosmic microwave background radiation and galaxy distributions.
- Enables analysis of light curves from supernova events to measure cosmic distances.
- Aids in recognizing dark energy effects by observing distant galaxies' photometric shifts.
The use of synthetic photometry in cosmological contexts extends to measuring the Hubble constant by applying techniques like the Tully-Fisher relation, which relates a galaxy's luminosity to its rotational velocity. Using synthetic analysis paired with observed magnitudes and redshifts:\[ v = H_0 d \]where \( v \) denotes the recession velocity and \( d \) is the distance, researchers refine this crucial cosmological parameter, contributing to deeper understanding of the universe's expansion rate.
synthetic photometry - Key takeaways
- Synthetic Photometry: A computational approach to simulate and analyze photometric properties of celestial objects using synthetic spectral data and theoretical modeling.
- Main Objective: Compare synthetic spectra with observations to understand physical properties like temperature and chemical composition of astronomical objects.
- Methodology Steps: Involves data collection, computational modeling, filter application, and analysis to compare with observational data.
- Techniques: Includes filter simulation, spectrum synthesis, and photometric calibration to match theoretical predictions with observations.
- Applications in Astronomy: Used to explore stellar and galactic spectra, calibrate photometric zero-points, and understand astronomical phenomena.
- Role in Physics: Enhances astrophysical research by detailing characteristics of stars and galaxies, and aids in cosmological studies and stellar population synthesis models.
Learn faster with the 12 flashcards about synthetic photometry
Sign up for free to gain access to all our flashcards.
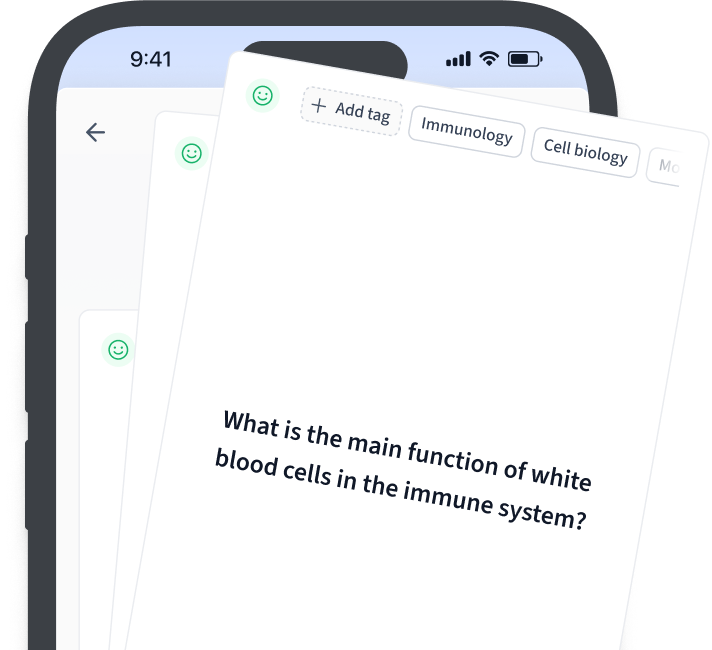
Frequently Asked Questions about synthetic photometry
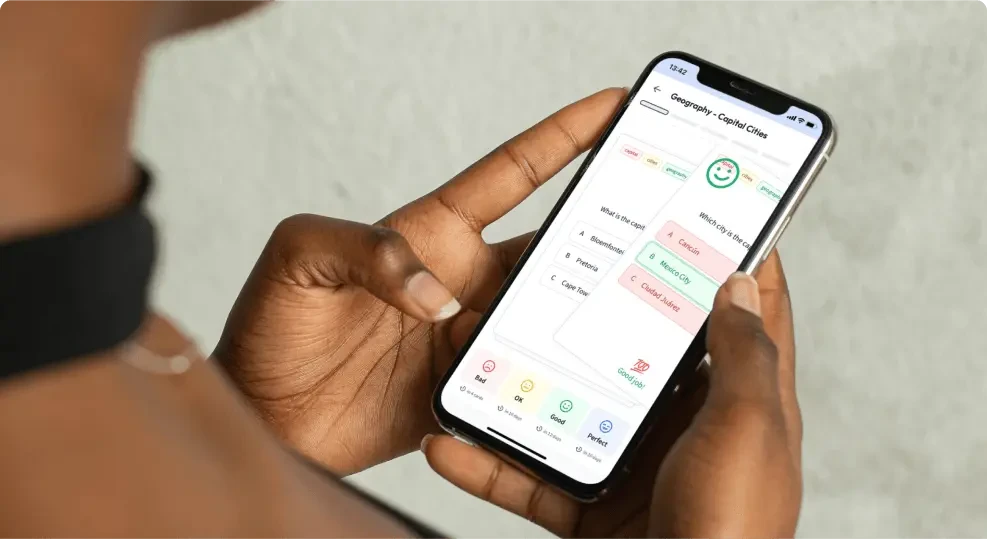
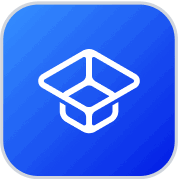
About StudySmarter
StudySmarter is a globally recognized educational technology company, offering a holistic learning platform designed for students of all ages and educational levels. Our platform provides learning support for a wide range of subjects, including STEM, Social Sciences, and Languages and also helps students to successfully master various tests and exams worldwide, such as GCSE, A Level, SAT, ACT, Abitur, and more. We offer an extensive library of learning materials, including interactive flashcards, comprehensive textbook solutions, and detailed explanations. The cutting-edge technology and tools we provide help students create their own learning materials. StudySmarter’s content is not only expert-verified but also regularly updated to ensure accuracy and relevance.
Learn more