Jump to a key chapter
What is Tidal Locking
Tidal locking is a fascinating celestial phenomenon that affects the gravitational interaction between a planet and its satellite or a star and its orbiting body. Understanding this phenomenon is crucial for comprehending how celestial bodies interact over time.
Definition of Tidal Locking
Tidal locking occurs when a celestial body's rotational period matches its orbital period around a partner body, resulting in the same side always facing its partner. This is due to gravitational forces acting over a prolonged period, causing synchronization of the two bodies' movements.
Explanation of Tidal Locking in Physics
The process of tidal locking can be explained through the interaction of gravitational forces between two celestial bodies. When a smaller body, like a moon, orbits a larger one, such as a planet, the gravitational pull causes deformation of the smaller body. Over time, this deformation leads to a redistribution of mass, eventually causing the smaller body to rotate at a speed that perfectly matches its orbital period.In physics, this phenomenon can be explained by considering the energy dissipation and angular momentum transfer between the two bodies. The gravitational forces create 'tidal bulges', which result in internal friction within the satellite. This gradual process dissipates the rotational energy of the satellite, causing it to lose either rotational velocity or synchronize its rotation with its orbital period.The fundamental equation describing tidal locking is:
- Gravitational potential energy: \((E_g = -\frac{G M_1 M_2}{r})\)
- Rotational kinetic energy: \((E_k = \frac{1}{2} I \omega^2)\)
- \(G\) is the gravitational constant,
- \(M_1\) and \(M_2\) are the masses of the two bodies,
- \(r\) is the distance between the centers of the two bodies,
- \(I\) is the moment of inertia of the body being locked,
- \(\omega\) is its angular velocity.
A common example of tidal locking is the Earth-Moon system. Our Moon is tidally locked to Earth, which means the same side of the Moon is always facing Earth. This is why you only ever see one side of the Moon from our planet.
Not all celestial bodies are tidally locked. For example, Earth is not tidally locked to the Sun, hence we have day-night cycles.
Over cosmic timescales, tidal locking is a common occurrence in planetary systems. Given enough time, the Earth itself may become tidally locked to the Sun, resulting in one side of the Earth permanently facing the Sun while the other remains in darkness. This process, however, would take billions of years. Tidal locking fundamentally depends on the mass and distance of the bodies involved, as well as the time scale over which the gravitational interactions occur.
How Tidal Locking Occurs
Understanding how tidal locking occurs will deepen your insight into the natural wonders of celestial mechanics. When you explore the physics behind this phenomenon, you unlock the mysteries of how gravitational forces preserve cosmic relationships over time.
Tidal Locking Phenomenon
The tidal locking phenomenon is intertwined with the laws of physics that govern gravitational interactions between celestial bodies. To grasp how this occurs, you must consider how energy dissipation and angular momentum affect a moon, planet, or star over time.
- The gravitational pull from a larger body induces tidal forces on the smaller body, leading to bulging on both sides of the smaller body.
- This gravitational influence causes a redistribution of mass and energy dissipation over time.
- The redistribution manifests as internal friction, converting rotational energy into heat.
- As energy is dissipated, the rotational speed slows until it matches the orbital period, achieving synchronization.
Gravitational potential energy: | \(E_g = -\frac{G M_1 M_2}{r}\) |
Rotational kinetic energy: | \(E_k = \frac{1}{2} I \omega^2\) |
- \(G\) is the universal gravitational constant.
- \(M_1\) and \(M_2\) represent the masses of the two interacting bodies.
- \(r\) is the distance between their centers.
- \(I\) is the moment of inertia, reflecting how mass is distributed relative to an axis.
- \(\omega\) is the angular rotation velocity of the body being locked.
Example: In the Earth-Moon system, the Moon is tidally locked to the Earth. This alignment results in only one face of the Moon being visible from Earth, illustrating the classic characteristic of tidal locking.
Mars' moon Phobos is expected to become tidally locked in the distant future due to similar gravitational mechanics.
Delving deeper into the universe, tidal locking demonstrates how time is an equally vital component in this synchronizing process. The timescale for a body to become tidally locked is considerably influenced by the distance between the bodies and their respective masses. The relationship governing this can be given by the lock timescale equation:\[ t_{lock} \approx \frac{a^6}{m_p R^5 Q} \frac{1}{m_s} \] where:
- \(t_{lock}\) represents the time required for locking to occur.
- \(a\) is the semi-major axis of the orbit.
- \(m_p\) is the mass of the planet,
- \(R\) the radius of the satellite,
- \(Q\) the quality factor, a measure of internal friction,
- \(m_s\) the mass of the satellite.
Examples of Tidal Locking
Tidal locking can be observed throughout the cosmos, providing compelling examples within our solar system and beyond. Each example helps illustrate the gravitational mechanics that lead to this fascinating phenomenon.
Tidal Locking in Our Solar System
In our solar system, tidal locking is a common phenomenon among moons and planets. The synchronous rotation that results from tidal locking can be observed in several key examples:
- The Moon and Earth: The most familiar example of tidal locking is our Earth-Moon system, where the Moon's rotation period matches its orbit around Earth, resulting in the same lunar face always facing our planet.
- Pluto and Charon: This system exemplifies mutual tidal locking, where both the dwarf planet Pluto and its moon Charon show the same face to each other constantly.
- Some of Jupiter's Moons: Moons such as Ganymede, Io, and Europa are tidally locked to Jupiter, ensuring they rotate synchronously with their orbital periods.
Deep diving into the gravitational intricacies, tidal locking occurs over extensive time periods. Different factors such as the mass of the bodies and distance significantly influence the timescale required for tidal locking. The equation for the tidal locking time is:\[ t_{lock} = \frac{a^6}{m_p R^5 Q} \frac{1}{m_s} \frac{1}{T^2} \]where:
- \(t_{lock}\) is the time needed for tidal locking.
- \(a\) is the orbital semi-major axis.
- \(m_p\) is the mass of the planet.
- \(R\) is the radius of the satellite.
- \(Q\) is the dissipation quality factor.
- \(m_s\) is the mass of the satellite.
- \(T\) reflects the gravitational torque.
Tidal Locking in Exoplanets
Beyond our solar system, tidal locking also occurs with exoplanets, orbiting stars at varying distances. Studying exoplanets and their potential tidal locking provides insights into their environmental conditions and the possibility of life.Many exoplanets within the habitable zone of red dwarf stars are likely tidally locked. Without axial rotation to distribute heat from the star, one side of the planet may experience perpetual daylight while the other remains in darkness. This significant temperature contrast can affect atmospheric circulation and thus, the potential for life.Some key examples of such exoplanets include:
- Proxima Centauri b: Orbiting in the habitable zone of its star, Proxima Centauri b may be tidally locked.
- TRAPPIST-1 System: Multiple planets in this system show likely tidal locking due to their close proximity to their parent star.
Importance of Tidal Locking in Astrophysics
Tidal locking plays a critical role in astrophysics by influencing the dynamics and development of planetary systems. It provides insight into the gravitational forces that bind celestial bodies, impacting both their physical characteristics and potential habitability.
Effects on Planetary Systems
In the realm of astrophysics, the effects of tidal locking on planetary systems provide crucial insights into how celestial bodies interact over time. By understanding these interactions, you can learn more about the long-term stability and evolution of these systems.
- Orbital Dynamics: Tidal locking ensures that a planet's rotation is influenced by its gravitational partner, affecting its orbital path around a star.
- Energy Dissipation: The process of achieving tidal locking involves energy dissipation as heat, which can lead to increased volcanic activity or tectonic movement.
- Atmospheric Influence: Tidal locking can lead to uneven heating of a planet's surface, with one side constantly exposed and the other in perpetual darkness. This affects atmospheric circulation and weather patterns.
- \(E_g\) is the gravitational potential energy.
- \(G\) is the gravitational constant.
- \(M_1\) and \(M_2\) are the interacting masses.
- \(r\) is the distance between their centers.
Example: The Earth-Moon system is a prominent example where tidal locking has stabilized Earth's gravitational interactions, significantly impacting tides and life cycles.
Note that tidal locking is a time-intensive process, requiring millions to billions of years for completion.
A deeper examination of tidal locking reveals its role in generating unique planetary characteristics. The redistribution of mass due to tidal forces is a gradual process impacting the moment of inertia and angular momentum. Consequently, the speed alterations follow a path defined by:\[ \tau = -\frac{2}{3} \frac{k m R^5 \omega}{Q a^6} \]where:
- \(\tau\) is the torque resulting from tidal forces.
- \(k\) is the Love number, representing the rigidity of the planet.
- \(m\) is the satellite mass.
- \(R\) the radius of the planet or moon.
- \(\omega\) the initial angular velocity.
- \(Q\) the dissipation factor, measuring internal energy loss.
- \(a\) the semi-major axis of orbit.
Implications for Habitability
The implications of tidal locking extend into the field of habitability, particularly for exoplanets potentially supporting life. Tidal locking influences environmental conditions and dictates the potential for life-sustaining atmospheres.Planets locked in synchronization might offer stabilized environments, but they also present challenges:
- Climate Stability: A tidally locked planet could experience vast temperature differences between its day and night sides, affecting atmospheric dynamics and hydrological cycles.
- Surface Conditions: Prolonged exposure to stellar radiation on the sunlit side could lead to deserts, while the dark side remains frigid.
Region | Climate Effect |
Sunlit Side | High radiation exposure, potential desertification |
Dark Side | Near-permanent ice coverage, hindered vegetation |
Terminator Line | Potentially habitable, balanced temperatures |
tidal locking - Key takeaways
- Tidal Locking Definition: Tidal locking occurs when a celestial body's rotational period matches its orbital period, resulting in one side permanently facing its partner.
- Gravitational Interaction: Tidal locking is caused by gravitational forces that lead to deformation and redistribution of mass, resulting in synchronized rotation.
- Energy Dissipation: Tidal locking involves energy dissipation and angular momentum transfer between celestial bodies, resulting in the conversion of rotational energy into heat.
- Equations: Key equations for understanding tidal locking involve gravitational potential energy and rotational kinetic energy.
- Examples: The Earth-Moon system is a classic example of tidal locking, and other well-known instances include Pluto and Charon, and some of Jupiter's moons.
- Long-term Effects: Over time, tidal locking can influence planetary systems' dynamics, energy dissipation, and even the habitability of exoplanets.
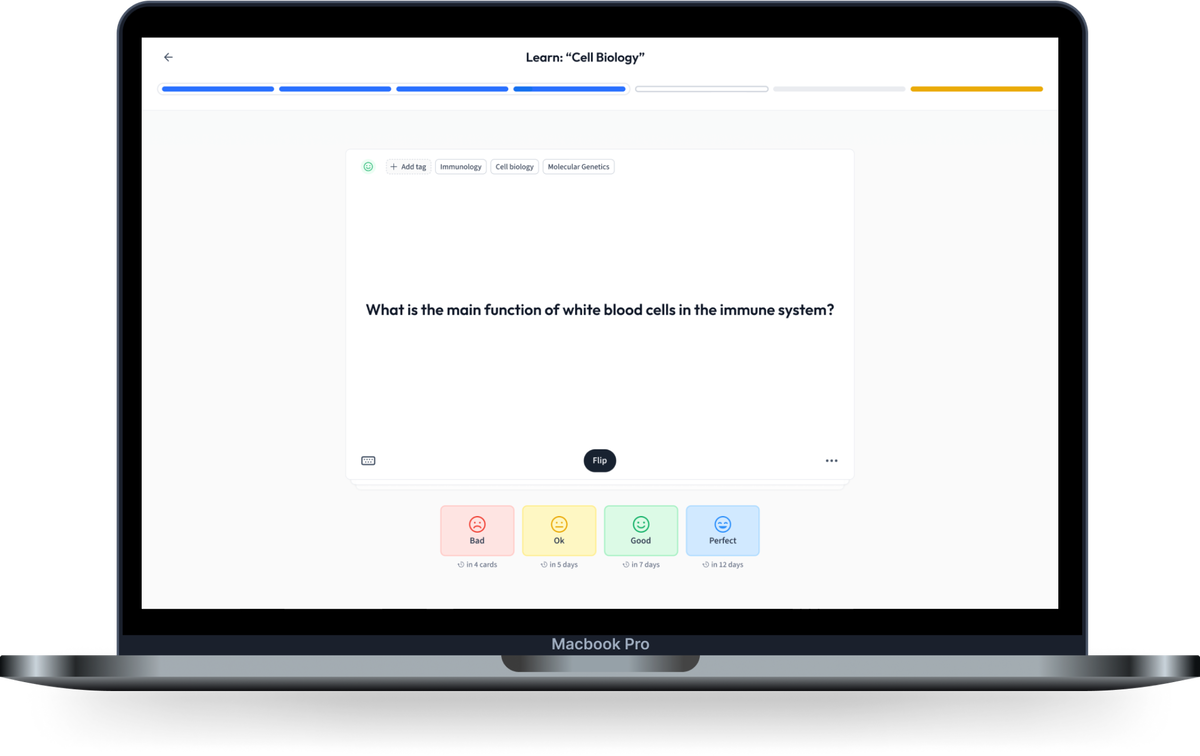
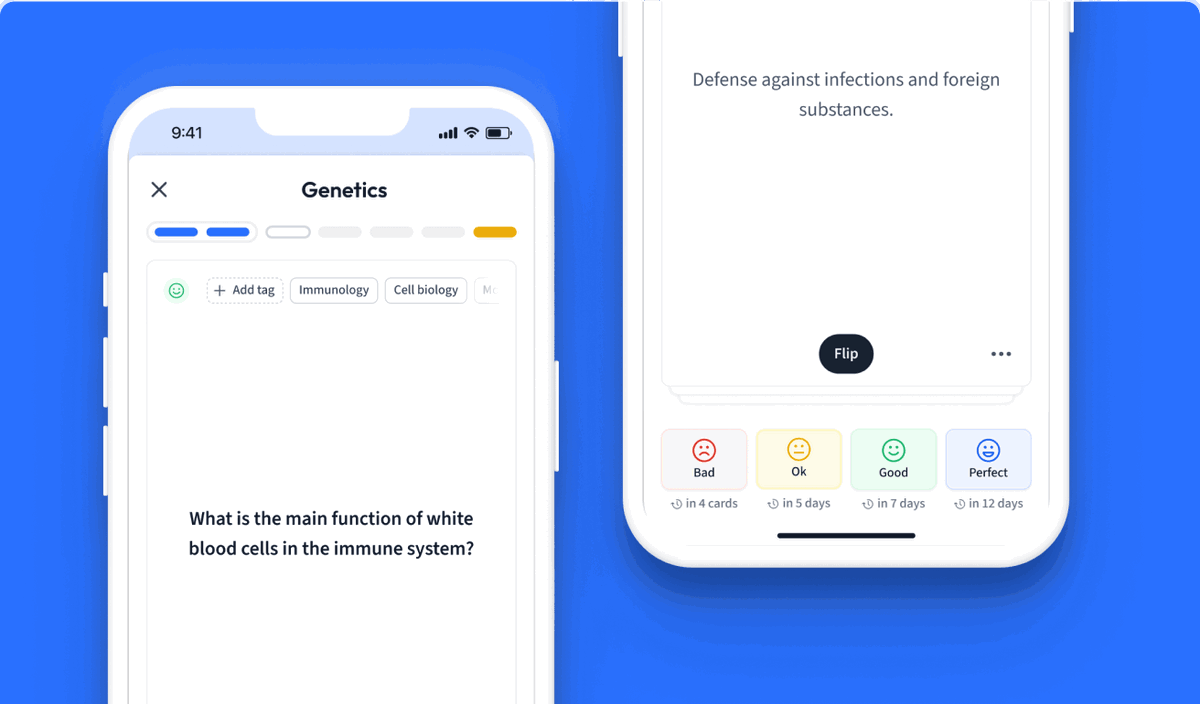
Learn with 12 tidal locking flashcards in the free StudySmarter app
Already have an account? Log in
Frequently Asked Questions about tidal locking
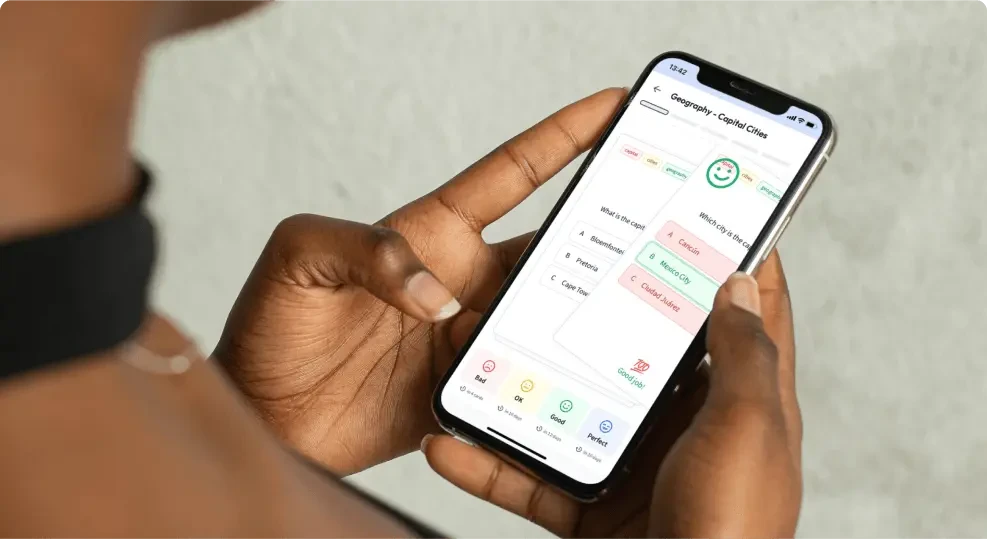
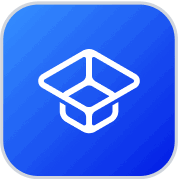
About StudySmarter
StudySmarter is a globally recognized educational technology company, offering a holistic learning platform designed for students of all ages and educational levels. Our platform provides learning support for a wide range of subjects, including STEM, Social Sciences, and Languages and also helps students to successfully master various tests and exams worldwide, such as GCSE, A Level, SAT, ACT, Abitur, and more. We offer an extensive library of learning materials, including interactive flashcards, comprehensive textbook solutions, and detailed explanations. The cutting-edge technology and tools we provide help students create their own learning materials. StudySmarter’s content is not only expert-verified but also regularly updated to ensure accuracy and relevance.
Learn more