Jump to a key chapter
White Dwarfs Explained
White dwarfs are fascinating remnants of stars that have reached the end of their life cycle. You might wonder why they occur and how they differ from other celestial bodies. Let's delve into the captivating characteristics and formation of these stellar phenomena.
What is a White Dwarf?
A white dwarf is a small, dense star that remains after a star has exhausted most of its nuclear fuel and shed its outer layers. These remnants typically have a mass equal to that of the sun but with a volume similar to that of Earth.
White dwarfs are composed primarily of electron-degenerate matter, which means they don't rely on nuclear fusion to support themselves. Instead, they withstand gravitational collapse due to quantum mechanical effects. Here are some key characteristics of white dwarfs:
- Mass: Typically around 0.5 to 1.4 solar masses.
- Volume: About equivalent to Earth.
- Temperature: High initially, cooling over time.
- Color: White when hot, fading as they cool.
White Dwarf Star Characteristics
White dwarfs are unique celestial objects with distinctive features that set them apart from other stars. They form from the remnants of medium-sized stars and exhibit remarkable characteristics, intricately linked to their intriguing life cycle.
Physical Characteristics of White Dwarfs
White dwarfs are notably **small** in size but **massive** in terms of density. Their physical characteristics include a wide range of temperatures and materials that affect their appearance. Here is a breakdown of their primary features:
- Mass: Generally ranges from 0.5 to 1.4 solar masses, adhering to the Chandrasekhar limit.
- Density: Extremely high, with masses comparable to the Sun compressed into a volume similar to Earth's.
- Temperature: Starts at around 100,000 Kelvin; they gradually cool, giving off a white or blue hue initially.
- Composition: Typically composed of carbon and oxygen due to the fusion processes of their progenitor stars.
The **Chandrasekhar limit** is the maximum mass (approximately 1.4 solar masses) that a stable white dwarf star can have before collapsing into a neutron star or black hole due to gravitational forces.
Imagine squeezing the sun’s mass into a space the size of Earth. The density would be such that a sugar-cube-sized amount of this material would weigh about as much as a car!
Mathematical Representations
Mathematics aids in understanding the structure and evolution of white dwarfs. One crucial expression is the **ideal gas law**, applicable to the degenerate electron gas in white dwarfs. It is given by:\[ PV = nRT \]However, in white dwarfs, **degeneracy pressure** plays a significant role instead of thermal pressure. The calculation of gravitational pressure balancing against this degeneracy pressure can be represented by the equation:\[ P = K \times \rho^{\frac{5}{3}} \]where P is the pressure, K is a constant, and ρ is the density. This equation shows why these stars remain stable despite the absence of thermal pressure.
Degeneracy pressure arises from the principles of quantum mechanics, specifically the Pauli exclusion principle, which prevents electrons from being packed into identical states.
The collapse of a star into a white dwarf typically occurs once its nuclear fusion processes cease, leaving behind the dense core. As fusion stops, the star’s outer layers expand and are shed, resulting in a planetary nebula and leaving a dense core. In certain cases, binary systems may give rise to supernovae if the white dwarf accretes enough mass from a companion star to exceed the Chandrasekhar limit, leading to a violent explosion. This fascinating process not only highlights stellar death but also contributes to the cosmic cycle of matter, seeding the interstellar medium with heavy elements.
White Dwarf Formation and Stellar Remnants
Understanding the formation of white dwarfs and their role as stellar remnants is crucial in astrophysics. These remnants are vitally important as they mark the final evolutionary stage of many stars, providing insights into both the past and future of cosmic development.
End Stages of Stellar Evolution
Stars like our Sun undergo a life cycle that concludes with the fascinating transition into a white dwarf. As stars deplete their nuclear fuel, they expand into red giants and eventually shed their outer layers. This process leaves behind a hot, dense core that cools and contracts into a white dwarf.As a star enters this phase, it expels its outer envelope, creating a planetary nebula. The remaining core becomes a **white dwarf**, no longer undergoing fusion reactions but maintaining equilibrium due to electron degeneracy pressure. The core's temperature initially remains high before it gradually cools over billions of years.
A planetary nebula refers to the cloud of gas and dust ejected from a star during its late-stage evolution, surrounding the newly formed white dwarf.
Consider our own Sun, which in approximately 5 billion years, will transform into a red giant, then ultimately become a white dwarf, encircled by a planetary nebula.
During the transformation into a white dwarf, the star loses much of its mass. Consequently, binary systems featuring a white dwarf and a companion star can experience mass transfer. In instances where the white dwarf accretes enough material from the companion to exceed the Chandrasekhar limit, a type Ia supernova may occur, a process vital to the distribution of heavier elements across the universe.
Structure of White Dwarfs
The internal structure of white dwarfs is distinct due to the predominance of electron-degenerate matter. These stars possess a pressure stemming from the Pauli exclusion principle, contributing to their stability. Here are some structural characteristics:
- Core: Composed primarily of carbon and oxygen, formed from prior helium fusion.
- Outer Shell: A thin layer, primarily hydrogen or helium.
- Pressure Support: Dominated by electron degeneracy rather than thermal pressure.
The high density of white dwarfs is so immense that a sugar-cube-sized amount of this material would weigh as much as a car!
White dwarfs, due to their extreme density and unique electron-degenerate state, are essential for understanding quantum mechanics in astronomical settings. They provide natural laboratories for studying fundamental physics, from relativity effects on massive stars to the quantum behavior of degenerate matter. Observations of white dwarfs can also help estimate the age of star clusters and, by extension, the universe itself.
white dwarfs - Key takeaways
- White Dwarf: A small, dense stellar remnant left after a star has depleted its nuclear fuel and shed its outer layers.
- White Dwarf Formation: Occurs when a star such as the Sun exhausts its nuclear fuel, expanding into a red giant, and leaves behind a hot, dense core as a white dwarf.
- Stellar Remnants: Remnants of stars in the end stages of evolution, primarily forming white dwarfs from medium-sized stars.
- Structure of White Dwarfs: Consists of a carbon and oxygen core, a thin outer shell of hydrogen or helium, with stability maintained by electron degeneracy pressure.
- End Stages of Stellar Evolution: Involves the transition from red giant to white dwarf, shedding outer layers to form a planetary nebula around the dense core.
- Degeneracy Pressure: Quantum mechanical pressure stabilizing white dwarfs and preventing gravitational collapse, described by density-dependent equations.
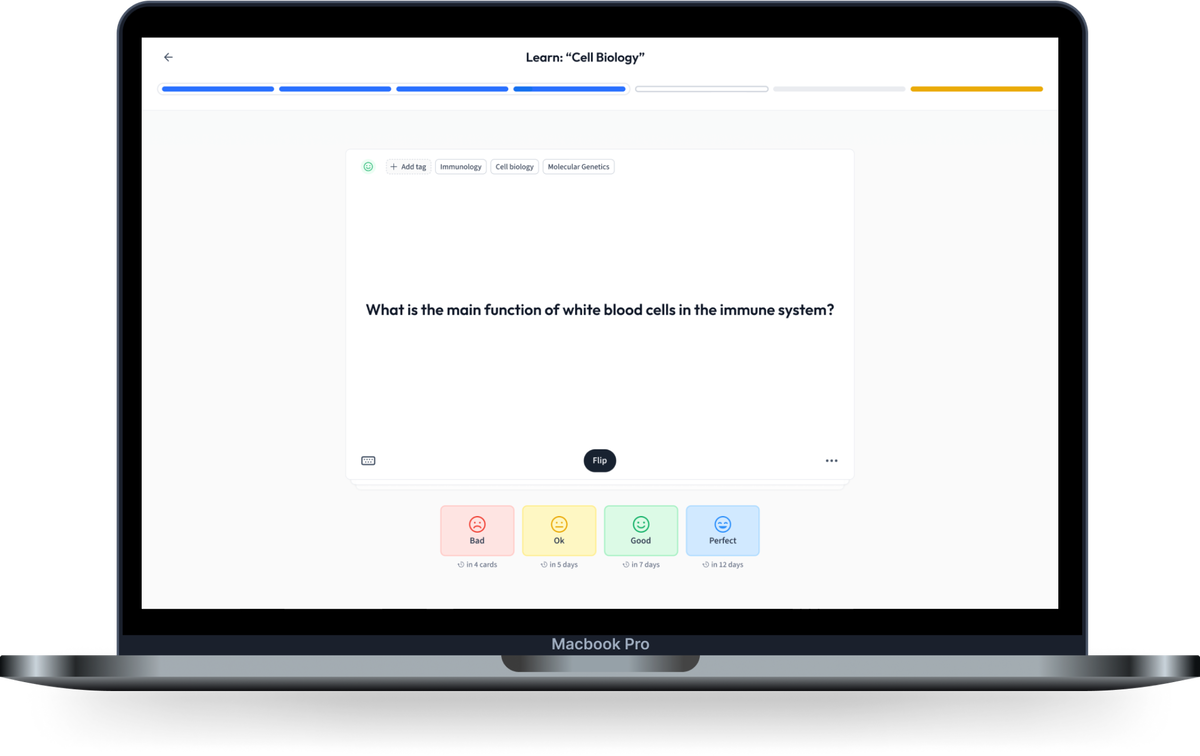
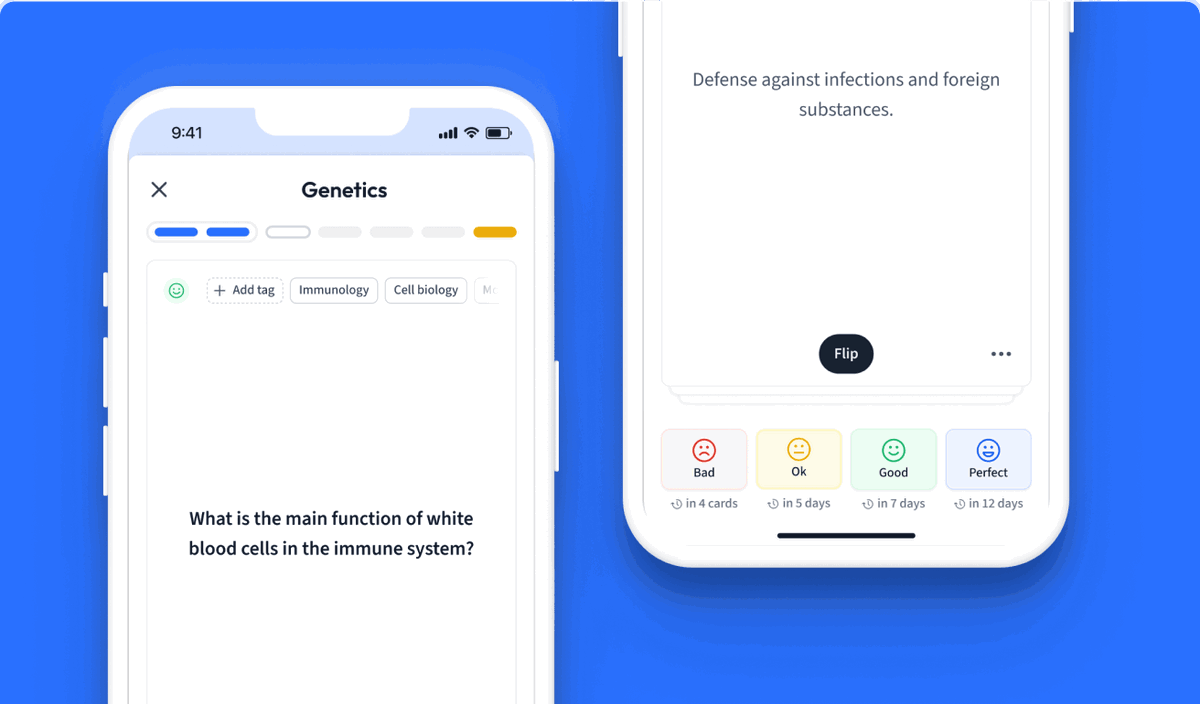
Learn with 12 white dwarfs flashcards in the free StudySmarter app
Already have an account? Log in
Frequently Asked Questions about white dwarfs
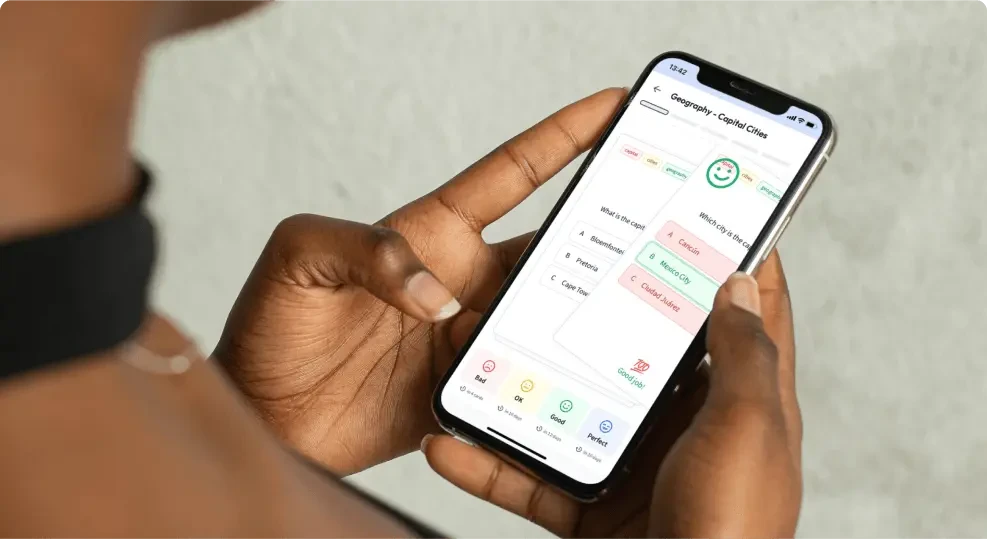
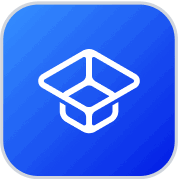
About StudySmarter
StudySmarter is a globally recognized educational technology company, offering a holistic learning platform designed for students of all ages and educational levels. Our platform provides learning support for a wide range of subjects, including STEM, Social Sciences, and Languages and also helps students to successfully master various tests and exams worldwide, such as GCSE, A Level, SAT, ACT, Abitur, and more. We offer an extensive library of learning materials, including interactive flashcards, comprehensive textbook solutions, and detailed explanations. The cutting-edge technology and tools we provide help students create their own learning materials. StudySmarter’s content is not only expert-verified but also regularly updated to ensure accuracy and relevance.
Learn more