Jump to a key chapter
What is X-Ray Astronomy
X-Ray Astronomy is a branch of astronomy that observes the universe in the X-ray part of the electromagnetic spectrum. X-ray astronomy allows you to study some of the most energetic phenomena in the universe, such as black holes, neutron stars, and supernovae.
Understanding the X-Ray Spectrum
X-rays are a form of electromagnetic radiation with wavelengths ranging from 0.01 to 10 nanometers, situated between ultraviolet light and gamma rays on the spectrum. In terms of energy, X-rays generally lie within the range of 0.1 to 100 keV (kiloelectronvolts). The study of these X-rays helps you detect cosmic sources that are otherwise invisible in other parts of the spectrum. The ability of X-ray astronomy to detect phenomena such as the accretion disks around black holes or the remnants of supernovae is unparalleled. This is because X-rays are produced in environments that are extremely high in temperature or have high-energy particle interactions.
X-Ray Astronomy: The study of astronomical objects at X-ray wavelengths, primarily involving high-energy, high-temperature events and phenomena.
Detecting X-Rays in Space
Since X-rays do not penetrate the Earth’s atmosphere easily, X-ray telescopes need to be placed aboard high-altitude balloons, rockets, or satellites. Several specialized missions and observatories contribute to this field:
- Chandra X-ray Observatory: Launched by NASA, it explores the universe through the window of X-ray light.
- XMM-Newton: A European Space Agency mission providing spectroscopic data on the X-ray universe.
- NuSTAR: The first focusing high-energy X-ray mission, providing images and information critical for understanding some of the most energetic objects in space.
Consider the case of a black hole. When matter falls into a black hole, it forms a swirling disk as it spirals inward, called an accretion disk. The frictional forces in this disk are immense, raising the temperature of the matter to millions of degrees and causing it to emit X-rays. By observing these X-rays, you can infer things like the mass and spin of the black hole based on the intensity and frequency distribution of the X-rays emitted.
Mathematics in X-Ray Astronomy
Mathematics plays a significant role in interpreting X-Ray astronomy data. For example, in analyzing X-ray emissions, you often use the Planck's Law, given as: Planck's Law: \[B(u, T) = \frac{2hu^3}{c^2} \frac{1}{e^{\frac{hu}{kT}} - 1}\]Where:
- \(B(u, T)\) is the spectral radiance
- \(h\) is Planck’s constant \(6.626 \times 10^{-34} J\cdot s\)
- \(u\) is the frequency
- \(T\) is the temperature
- \(c\) is the speed of light
- \(k\) is Boltzmann's constant \(1.381 \times 10^{-23} J/K\)
Imagine you are studying the distribution of X-ray brightness across a galaxy cluster. By mapping this distribution, you can learn about the mass and gravitational potential of the cluster's dark matter, an invisible component that shapes the structure of the universe. This mapping is possible using mathematical techniques like the application of Poisson statistics to model the distribution of X-ray photons across the sky. Specifically, the equation for Poisson statistics is given as: \[ P(k; \lambda) = \frac{e^{-\lambda} \lambda^{k}} {k!} \]Where
- \(P(k; \lambda)\) represents the probability of \(k\) events in an interval
- \(\lambda\) represents the average number of times event occurs per interval
X-Ray Astronomy Definition and Techniques
X-Ray Astronomy offers a vital perspective on understanding high-energy events in the universe. Observing X-rays from space requires special instrumentation, as Earth's atmosphere blocks these rays from reaching the surface.
Characteristics of X-Ray Sources
X-ray sources vary in nature, ranging from stars and galaxies to exotic objects like black holes and neutron stars. The measurement of X-ray emissions provides insights into:
- The behavior of matter at extreme conditions
- The dynamics of cosmic phenomena
- The chemical composition of astronomical bodies
X-Ray Emission: The release of X-ray photons from a source, typically generated by high-energy processes.
For instance, within a neutron star system, the companion star's material spirals onto the neutron star, forming an accretion disk. The immense gravitational energy of the neutron star heats the material to high temperatures, emitting X-rays. This process reveals the mass transfer and other complex interactions through the emitted X-rays, providing a detailed example of X-ray production.
Techniques for Observing X-Rays
Observing X-rays requires unique techniques, as regular optical or radio telescopes cannot capture X-ray wavelengths. Key techniques involve:
- ***X-ray Telescopes***: Use focusing techniques like grazing incidence mirrors to redirect and concentrate X-rays onto detectors.
- Space-Borne Observatories: Satellites equipped with X-ray detecting technology bypass Earth's atmosphere to capture X-ray data, like NASA's Chandra Observatory.
The mathematics behind X-ray detection frequently involves analyzing the energy flux as detected in an X-ray telescope’s imaging system. For instance, the equation for calculating photon energy is:\[ E = hf \]Where:
- \(E\) is the energy of the photon
- \(h\) is Planck's constant (\(6.626 \times 10^{-34} \text{ J\cdot s}\))
- \(f\) is the frequency of the X-ray
X-ray astronomers often collaborate with researchers in other wavelengths to create a multi-wavelength view of the universe, providing a more complete picture of astronomical events.
X-Ray Astronomy Facts
X-Ray Astronomy opens up a window to some of the universe's most energetic processes. By studying X-rays, you can gain valuable insights into phenomena that are not observable through other types of electromagnetic radiation.
X-Ray Astronomy Instruments
To observe X-rays, astronomers use specially designed instruments often located on satellites, beyond Earth's atmosphere. These instruments include:
- X-Ray Telescopes: These employ grazing incidence optics to focus X-rays onto detectors, allowing detailed study of X-ray sources.
- Charge-Coupled Devices (CCDs): These detectors convert incoming X-rays into electronic signals which are then processed to create images and spectra.
The technique of grazing incidence optics is critical in X-ray astronomy. Due to the penetrating nature of X-rays, traditional lenses cannot focus them. Instead, mirrors reflect X-rays at shallow angles, converging them onto detectors. This principle can be expressed using the angle of incidence, \(\theta_i\), and the angle of reflection, \(\theta_r\), where \theta_i = \theta_r\.
Applications of X-Ray Astronomy
X-ray astronomy has several important applications, including:
- Black Holes: Observing accretion disks around black holes in X-rays reveals information about their gravitational fields and matter infall rates.
- Neutron Stars: X-ray emissions from neutron stars help determine their magnetic field strength and rotational behavior.
- Supernova Remnants: Studying the X-rays from these remnants helps analyze the composition and structure of expelled materials.
Consider how X-rays are used to study a supernova remnant. By observing the X-ray spectrum, you can identify elements such as oxygen, magnesium, and iron, providing clues about the explosion process and the progenitor star's composition.
Supernova Remnant: The remaining structure and expanding shockwave of a star that has exploded in a supernova.
Mathematical Framework of X-Ray Observations
Analyzing X-ray data requires complex mathematics. For example, you might calculate the surface temperature of a neutron star using a form of the Stefan-Boltzmann Law: \[ L = 4\pi R^2\sigma T^4 \]Where:
- \(L\) is the luminosity
- \(R\) is the radius of the emitting area
- \(\sigma\) is the Stefan-Boltzmann constant
- \(T\) is the surface temperature
X-ray astronomy also provides vital information on galaxy clusters, shedding light on dark matter through the observation of the hot gas in these massive conglomerates.
X-Ray Astronomy Satellites and Technologies
Satellites and advanced technologies play a pivotal role in X-Ray Astronomy, providing the necessary means to observe and analyze X-rays from outer space. These technologies allow for unprecedented exploration of the cosmos, helping you understand some of the most dynamic and energetic processes in the universe.
Importance of X-Rays in Astronomy
X-rays offer crucial insights into high-energy astrophysical phenomena. Key observations can be made only through X-ray astronomy, shedding light on:
- Cosmic structures such as galaxies and black holes
- Specific stellar events like supernovae and star formation
- Interactions in binary star systems
Binary Star System: A system of two stars orbiting around their common center of mass.
Consider the study of a binary star system where one star is a neutron star. Due to its powerful gravitational pull, it attracts material from its companion star, forming an accretion disk that releases X-rays. By examining the X-ray emissions, you can infer properties such as mass transfer rates and orbital period.
X-ray astronomy often complements other astronomical observations to create a comprehensive picture of astronomical phenomena, enhancing multi-wavelength studies.
Advancements in X-Ray Astronomy Techniques
Several advancements have driven the field of X-ray astronomy forward, including the development of better detectors and imaging technology. Notable advancements are:
- Improved X-ray Mirrors: These use multi-layer coatings to enhance reflection efficiency.
- High-Resolution Spectroscopy: Enables precise measurement of X-ray energies to study physical conditions in various environments.
An exciting advancement in X-ray observation techniques is the introduction of Microcalorimeter Spectroscopy. This method measures the slight temperature rise when X-rays are absorbed by a detector, allowing for precise energy measurements. The process can be mathematically analyzed as follows:\[ \Delta E = kT \log \left( 1 + \frac{\Delta T}{T} \right) \]Where:
- \(\Delta E\) is the energy resolution
- \(k\) is Boltzmann's constant
- \(T\) is the initial temperature
- \(\Delta T\) is the change in temperature
x-ray astronomy - Key takeaways
- X-Ray Astronomy Definition: The study of astronomical objects using X-rays, focusing on high-energy, high-temperature events like black holes and supernovae.
- X-Ray Spectrum: X-rays range from 0.01 to 10 nanometers in wavelength and 0.1 to 100 keV in energy, positioned between ultraviolet light and gamma rays.
- Detection Techniques: X-rays are observed using space-born telescopes and satellites, such as the Chandra X-ray Observatory and XMM-Newton, to overcome Earth's atmospheric interference.
- Instruments and Techniques: Utilize grazing incidence optics and Charge-Coupled Devices (CCDs) to measure X-ray emissions, offering insights into energetic cosmic phenomena.
- Mathematical Applications: Involve the use of Planck's Law, Poisson statistics, and energy calculations to interpret X-ray observations and understand celestial bodies.
- Importance of X-Ray Astronomy: Reveals crucial information on cosmic structures, stellar events, and high-energy processes, enhancing the understanding of the universe's mechanics.
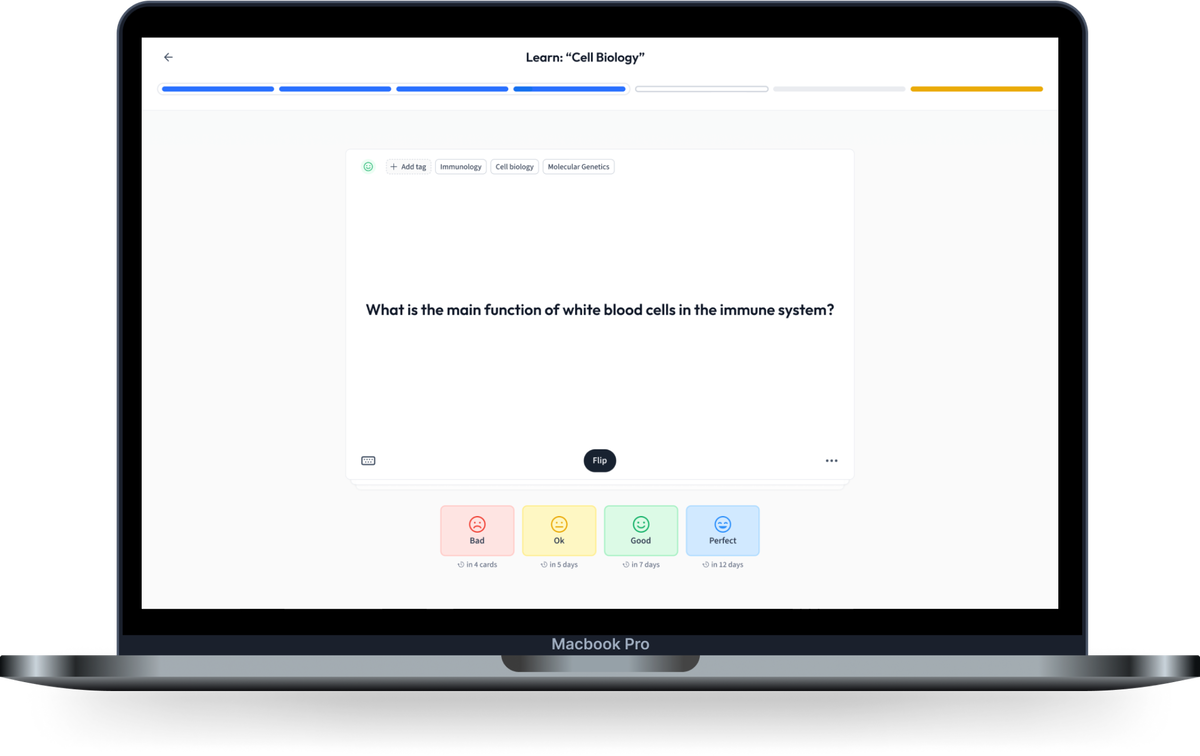
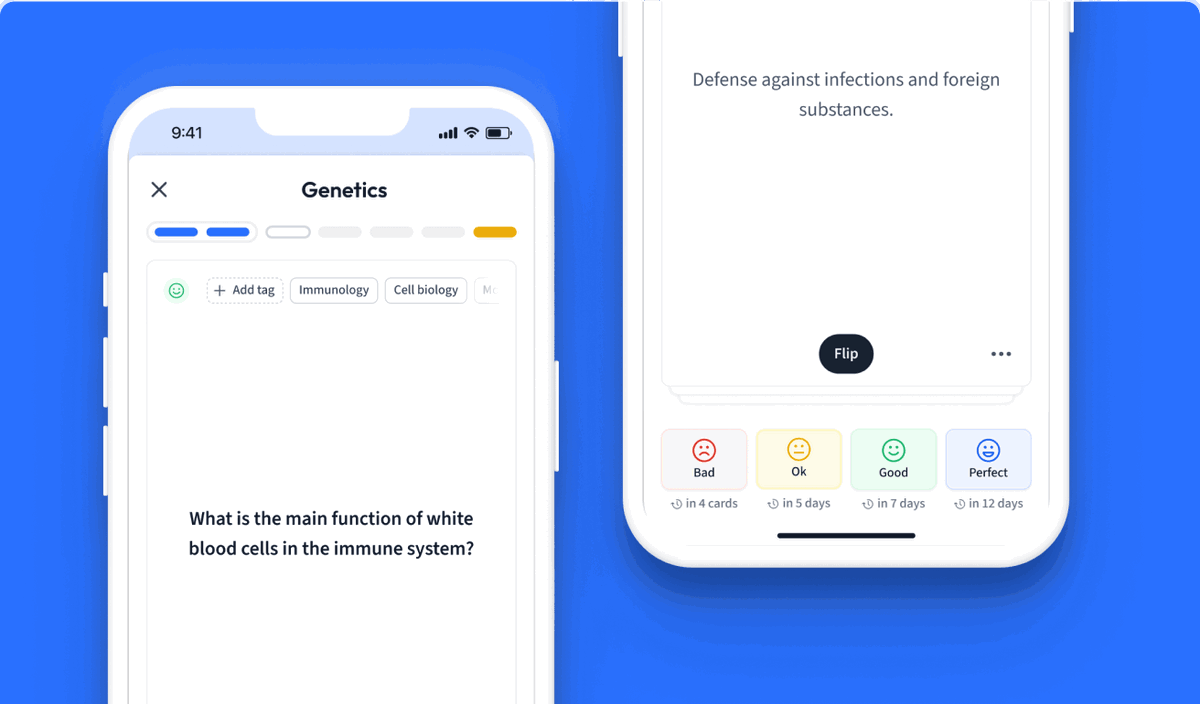
Learn with 12 x-ray astronomy flashcards in the free StudySmarter app
Already have an account? Log in
Frequently Asked Questions about x-ray astronomy
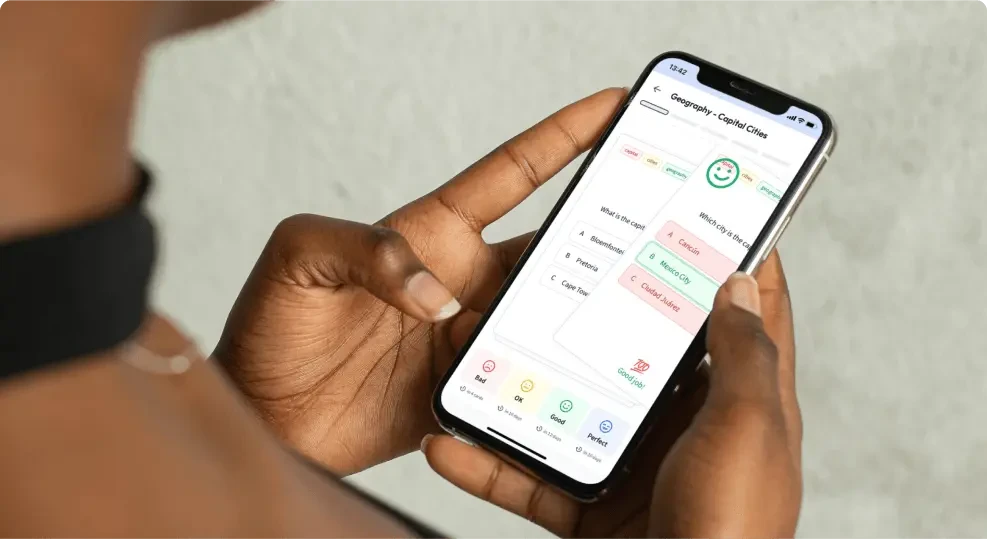
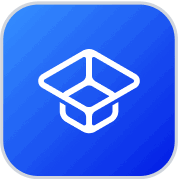
About StudySmarter
StudySmarter is a globally recognized educational technology company, offering a holistic learning platform designed for students of all ages and educational levels. Our platform provides learning support for a wide range of subjects, including STEM, Social Sciences, and Languages and also helps students to successfully master various tests and exams worldwide, such as GCSE, A Level, SAT, ACT, Abitur, and more. We offer an extensive library of learning materials, including interactive flashcards, comprehensive textbook solutions, and detailed explanations. The cutting-edge technology and tools we provide help students create their own learning materials. StudySmarter’s content is not only expert-verified but also regularly updated to ensure accuracy and relevance.
Learn more