Jump to a key chapter
Understanding Non Ohmic Conductor
Welcome, budding physicists! Today, you are going to step into an intriguing segment of electricity that revolves around the concept of Non Ohmic Conductors. Are you up for the challenge?Define Non Ohmic Conductor
Let's not keep you waiting any longer! A Non Ohmic Conductor, as intriguing as it sounds, is a little different from what it seems. To make it simpler, let's first consider an Ohmic conductor. An Ohmic conductor obeys Ohm's Law, which states that the current \(I\) is directly proportional to the voltage \(V\). In simple words, \[ V = I \cdot R \] Where:- \(V\) is the voltage applied across the conductor
- \(I\) is the current flowing through the conductor, and
- \(R\) is the resistance of the conductor.
If a conductor follows this law, it's termed as an Ohmic conductor, i.e., the voltage-current graph is linear.
Non Ohmic Conductor Meaning Explained
In a Non Ohmic Conductor, the relationship differs due to various factors like temperature, voltage, the material of the conductor, etc. This means with a change in these parameters, the current doesn't change in accordance with Ohm's Law.Consider an LED (Light Emitting Diode). It does not obey Ohm's law at all ranges of electric current. If graphed, it presents a curve instead of a straight line, indicating it is, indeed, a Non Ohmic Conductor.
Material | Ohmic or Non Ohmic Conductor |
Copper | Ohmic |
Silicon | Non Ohmic |
LED | Non Ohmic |
The Science behind Non Ohmic Conductor
Now that you have a basic understanding of Non Ohmic Conductors, let's dive a little deeper.In metals, the electrons serve as the charge carriers. As you increase the voltage, you essentially increase the kinetic energy of these electrons. The increased kinetic energy causes them to vibrate more rapidly (which contributes to a rise in temperature), thus disrupting the constant rate of increase in current.
Examples of Non Ohmic Conductors in Physics
While Non Ohmic Conductors may seem like an obscure topic limited to the realm of lab experiments and theoretical physics, the truth is that these kinds of conductors are quite prevalent and play a significant role in technology and everyday life. The operation of many devices, including transistors and diodes, highly depends on the non-ohmic behaviour of specific materials.Common Examples of Non Ohmic Conductors
Starting with the most commonly known example of Non Ohmic Conductors - the semiconductor family. Semiconductors, such as Silicon and Germanium, are not linear resistors. This is mostly due to the fact that as voltage increases, these materials tend to release more charge carriers, disrupting the traditionally linear relationship between current and voltage. A drawn-out graph of current to voltage for either of these materials would yield an S-like curve, not a straight line as Ohm’s Law would predict. Another notable example is made up of filament lamps. The relationship between voltage and current in a filament lamp is far from linear, mostly due to the fact that as more current flows through the lamp’s filament, its temperature increases. As the temperature escalates, so does the filament’s resistance, leading to a non-linear relationship between voltage and current.Thermistors are yet another example of Non Ohmic Conductors. These devices are typically used in temperature sensors, as their resistance is highly dependent upon temperature, violating the linearity of Ohm’s Law.
Non Ohmic Conductor Instances in Everyday Life
Non Ohmic Conductors are not just limited to laboratories and theoretical analysis. They permeate everyday life in various ways, many of which you may not be aware. From light bulbs to microwaves to touchscreen devices, these conductors are everywhere. For instance, a toaster is a classic example of a Non Ohmic Conductor. The heating element inside the toaster, generally made of a metal alloy, tends to get red-hot, thus increasing its temperature. This, in turn, increases its resistance, leading to a non-linear curvature on the graph of voltage versus current. Another example that's ever-present in modern life is the LED (Light Emitting Diode). The LEDs in your computer screen or television work based on non-ohmic principles. When minimal voltage is applied, they emit no light. Still, with an increase in voltage, the emitted light intensifies, demonstrating the non-linear relationship between current and voltage. Indeed, Non Ohmic Conductors are all around us - you just have to know where to look! And now that you understand them better, hopefully, the world around you will seem just a little bit more interesting - or at least, more scientifically complex.Characteristics of a Non Ohmic Conductor
Non Ohmic Conductors, as you've already discovered, deviate from Ohm's law and offer a myriad of intriguing characteristics that make them uniquely useful in various applications. From semiconductors to filament bulbs, the characteristics of a Non Ohmic Conductor make for an interesting analysis.Fundamental Characteristics of Non Ohmic Conductor
The fundamental characteristics of a Non Ohmic Conductor primarily relate to its interaction with different voltages. Here are some key features: 1. Non-linear Voltage-Current Relationship: As stated earlier, Non Ohmic Conductors don't necessarily follow Ohm's law that dictates a linear relationship between voltage and current. Instead, these conductors exhibit a non-linear graph with a curve, rather than a straight line. The graph can take an S shape, bell shape, or even exponential growth, depending largely on the specific material, operating conditions, and measurement techniques used. 2. Temperature Dependence: The behaviour of these Non Ohmic Conductors is intricately linked with temperature. As temperature rises, the resistance in these conductors tends to deviate from a constant level. It either drops (as in case of negative temperature coefficients like thermistors) or rises (for positive temperature coefficients like metal filament lamps). 3. Material-Specific Behaviour: Non Ohmic Conductors display a wide and diverse array of reactions to voltage and temperature changes, largely dependent on the material. Semiconductors like germanium and silicon produce an S-shaped curve, filament lamps offer a parabolic curve, and thermistors show exponential growth or decline, all within non-linear parameters. 4. Dissipation of Energy: Segueing from these above traits is another key characteristic – dissipation of energy. Non Ohmic Conductors lose more energy in the form of heat because of their nonlinear relationship between voltage and current. This significant energy dissipation can be both an asset, like in heating devices, or a disadvantage, such as in circuits where energy efficiency is critical.Double direction conductivity: In some particular cases, like diodes (a subset of which are LEDs), these Non Ohmic Conductors exhibit Directional conductivity. This means that they conduct electricity more efficiently in one direction than they do in the opposite direction.
Non Ohmic Conductor Characteristics and their Implications
The unique characteristics of Non Ohmic Conductors have far-reaching implications, shaping many aspects of modern technology and electronics. Primarily an increased understanding of these specific characteristics has allowed for a more considered selection of materials in applications across the electronic and technological world. For example, semiconductors like Silicon and Germanium are chosen for their specific non-ohmic characteristics – precisely that directionality in electricity conduction – and used extensively in diodes and transistors. These Non Ohmic Conductors have been foundational to the microelectronics revolution, finding application in virtually all electronic appliances of the digital age--from microprocessors to databanks. Thermistors, with their temperature-dependent resistance, have provided excellent utility as temperature sensors in thermometers, automotive fuel injection systems, or home appliances like toasters and irons. Energy dissipation characteristics have been harnessed in electric heating appliances, like ovens, toasters, etc., where large amounts of heat need to be created effectively. Importantly, these characteristics have not only taught us about the essential physics of electrical conductance but also enabled vital breakthroughs in technology, ensuring the utility of these conductors in our everyday life. While this only scratches the surface of the complex world of Non Ohmic Conductors, understanding their fundamental characteristics is a vital first step to harness the power of these resistive devices in electronics and technology.Dealing with Resistance in Non Ohmic Conductors
Dealing with resistance in Non Ohmic Conductors tends to be a little more challenging than dealing with resistance in Ohmic Conductors. Why so? The reason lies in the inherent properties of non-ohmic conductors, such as their non-linear voltage-current relationship, temperature, material properties, and energy losses, as decoded previously.How to Find the Resistance of a Non Ohmic Conductor
Unlike ohmic conductors, the resistance of a non-ohmic conductor doesn't stay constant when the applied voltage or current varies. This leads to a non-linear relationship between voltage and current. Typically, the resistance of a Non Ohmic Conductor is found through a method known as the "slope-method". The resistance is equal to the change in voltage divided by the change in current, much akin to the slope of a line on a graph. However, as the graph isn’t a straight line, differentiating two points on the graph gives different resistances. To put it mathematically, the resistance is calculated as \( R = \frac{{ΔV}}{{ΔI}} \). Where \( R \) is the resistance, \( ΔV \) is the change in voltage and \( ΔI \) is the change in current. As you approach a particular voltage or current value, this definition morphs into a local description of resistance or "differential resistance", as it is often called, defined as \( R = \frac{{dV}}{{dI}} \).In many practical non-ohmic devices such as diodes, varistors, or thermistors, resistance (or rather, differential resistance) can vary dramatically over different ranges of applied voltage or current, often by orders of magnitude. The capability to predict and strategically deploy this resistance variation is what enables such devices to function optimally in their respective roles, from surge protection to temperature sensing.
Factors Impacting Resistance in Non Ohmic Conductors
Determining resistance in Non Ohmic Conductors isn't just about calculating differences in voltage and current. There's more to the story! Variable factors like material properties, temperatures, the intensity of the electric field, and the sort of doping on semiconductors, all can have a massive influence on the resistance. 1. Material Properties: As stated, the material of the conductor plays a role. Depending on which atoms make up the material, the number of free electrons available to move and conduct electricity varies, thus impacting resistance. For example, semiconductor elements introduce a myriad of atoms integrated into the material, causing free electrons to collide and thus create resistance. 2. Temperature: Resistance can increase or decrease with varying temperatures, and this is often a broader characteristic of Non Ohmic Conductors. An increase in heat can cause atoms within a conductor to vibrate more, leading to higher resistance by offering more collisions and thereby slowing the passage of electrons. 3. Intensity of Electric Field: In semiconductors, a strong electric field can generate a higher quantity of charge carriers, subsequently inducing a lower resistance state. This field-dependent resistance can prove critical in devices such as varistors that under a normal electrical field bode high-resistance but in a high-intensity electrical field, their resistance drops, acting as a pathway for excess voltage and protecting electronic items from power surges. 4. Doping: In regards to semiconductors, doping or adding impurities can greatly impact the resistance. More doping equals more free electrons, thus reducing resistance. Through controlling the amount of these impurities, manufacturers can control the resistance in semiconductor devices and optimize their performance.While analyzing the resistance of semiconductors (a typical Non Ohmic Conductor), temperature plays a role: For intrinsic semiconductors, when temperatures soar, the semiconductor material obtains thermal energy and employs it to push more electrons into the conduction band, therefore leading to lower resistance.
Broadening your Knowledge about Non Ohmic Conductors
Broadening your understanding of Non Ohmic Conductors can be a leap towards comprehending complex electronics and the role these unique conductors play. From devices in your everyday life to sophisticated hardware in the tech industry, Non Ohmic Conductors are all around you, and it helps to know them better.Frequently Asked Questions about Non Ohmic Conductors
You might have several questions about Non Ohmic Conductors as this topic can be a bit confusing. Here are some common queries with detailed answers: 1. Why do Non Ohmic Conductors not follow Ohm's Law? Non Ohmic Conductors don't follow Ohm's law because their resistance isn't constant. It changes with current or voltage changes, displaying a non-linear relationship between voltage and current. This often involves variations in temperature, material specifics, and energy dissipation. 2. What devices incorporate Non Ohmic Conductors? Many devices utilise Non Ohmic Conductors, such as filament bulbs, diodes, transistors, thyristors, varistors, and thermistors. 3. How do temperature changes influence Non Ohmic Conductors? Temperature changes can have a significant effect. When temperature rises, the resistance either drops (for negative temperature coefficients) or rises (for positive temperature coefficients), hence leading to non-linear current-voltage characteristics. 4. Can Non Ohmic Conductors be used efficiently despite their energy losses? Yes, Non Ohmic Conductors can be used efficiently despite their inherent energy losses. Devices like resistors in circuits limit current flow, filament lamps provide light, and surge protectors safeguard electrical appliances by utilising energy loss effectively.Clarifying Common Misunderstandings about Non Ohmic Conductors
In the world of Physics, misconceptions abound, particularly regarding complex subjects like Non Ohmic Conductors. Let's clear up a few common misunderstandings: 1. Misunderstanding: Non Ohmic Conductors are inefficient due to high energy loss. Clarification: While Non Ohmic Conductors do lose more energy in the form of heat compared to their Ohmic counterparts, this isn't a disadvantage in every scenario. For instance, in heating equipment or light sources like lamps, this energy is harnessed for useful output. Thus, these conductors are efficient within their prescribed applications. 2. Misunderstanding: Non Ohmic Conductors always have a negative temperature coefficient. Clarification: This is incorrect. Although some Non Ohmic Conductors like thermistors exhibit a negative temperature coefficient (their resistance decreases as temperature increases), this is not a rule for all. Others, like filament lamps, have a positive temperature coefficient: their resistance increases with temperature. 3. Misunderstanding: The resistance of Non Ohmic Conductors is incredibly difficult to determine. Clarification: It might seem intimidating because the resistance is not constant and depends on factors like temperature and the voltage or current applied. However, by graphing the voltage-current relationship and employing the "slope-method", or considering a local description of resistance, the process becomes manageable. Hopefully, confrontations with this factual information have helped clear up some of the common misconceptions about Non Ohmic Conductors, helping to build your understanding of this fascinating topic even further.Non Ohmic Conductor - Key takeaways
- A Non Ohmic conductor is characterized by a non-linear voltage-current relationship, indicating that resistance in these conductors changes with current or voltage changes. This non-linearity makes it deviate from Ohm's law.
- Examples of Non Ohmic Conductors include semiconductors such as Silicon and Germanium, filament lamps, and thermistors. Their behaviour varies with temperature and voltage leading to a non-linear graph when drawn between voltage and current.
- Fundamental characteristics of a Non Ohmic Conductor include non-linear voltage-current relationship, temperature dependence, material-specific behaviour, energy dissipation, and double directional conductivity in some cases like diodes.
- The resistance of a Non Ohmic Conductor is found through a method known as the "slope-method". It is calculated as R = ΔV/ΔI, where R is the resistance, ΔV is the change in voltage and ΔI is the change in current. This method defines the local description of resistance or "differential resistance".
- Factors that impact resistance in Non Ohmic Conductors include material properties, temperatures, the intensity of the electric field, and the level of doping in semiconductors.
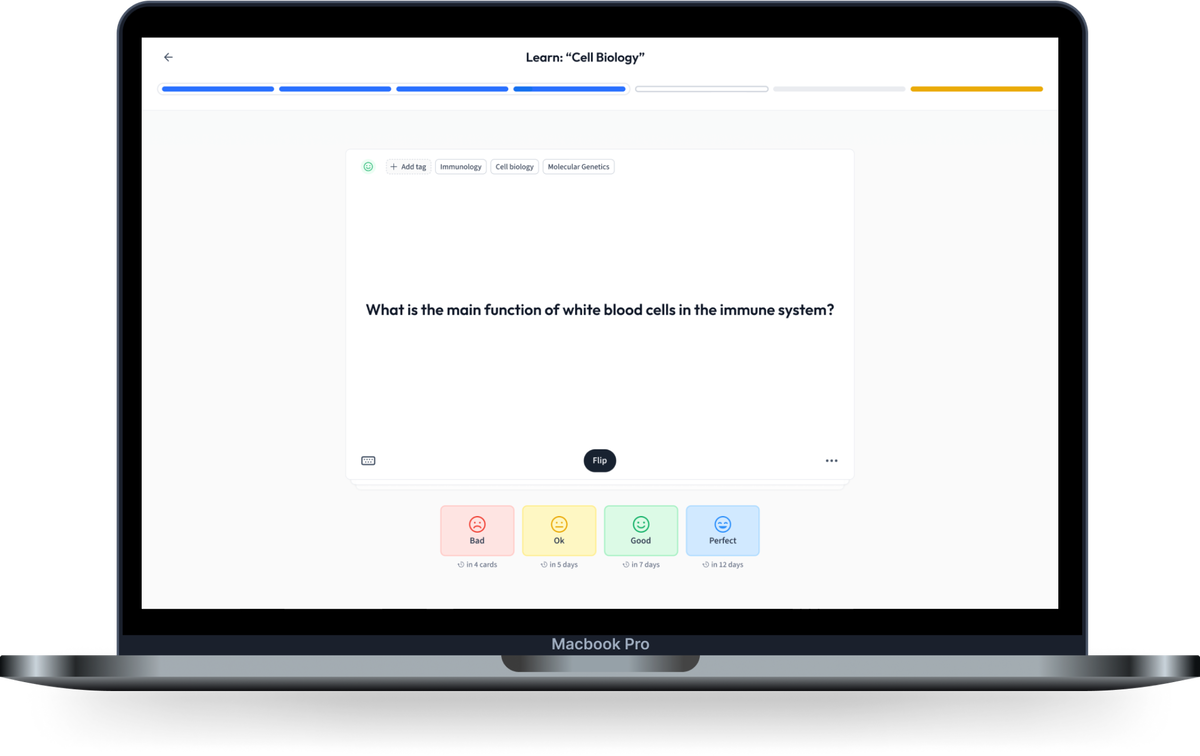
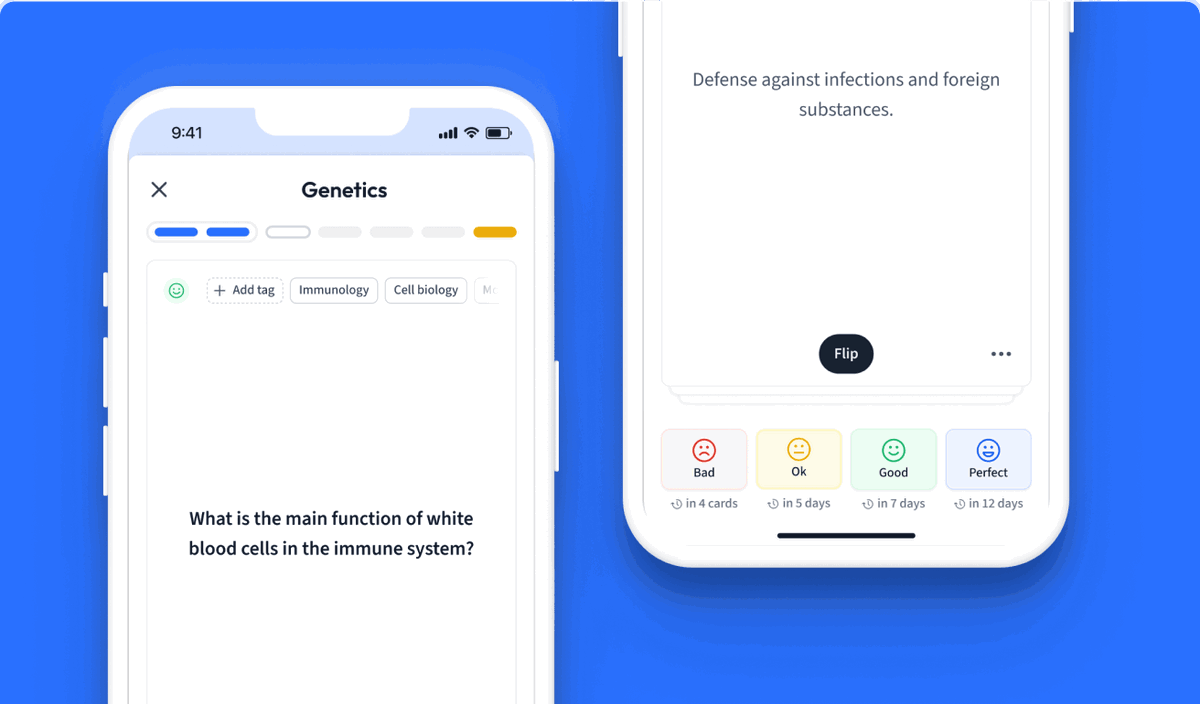
Learn with 15 Non Ohmic Conductor flashcards in the free StudySmarter app
We have 14,000 flashcards about Dynamic Landscapes.
Already have an account? Log in
Frequently Asked Questions about Non Ohmic Conductor
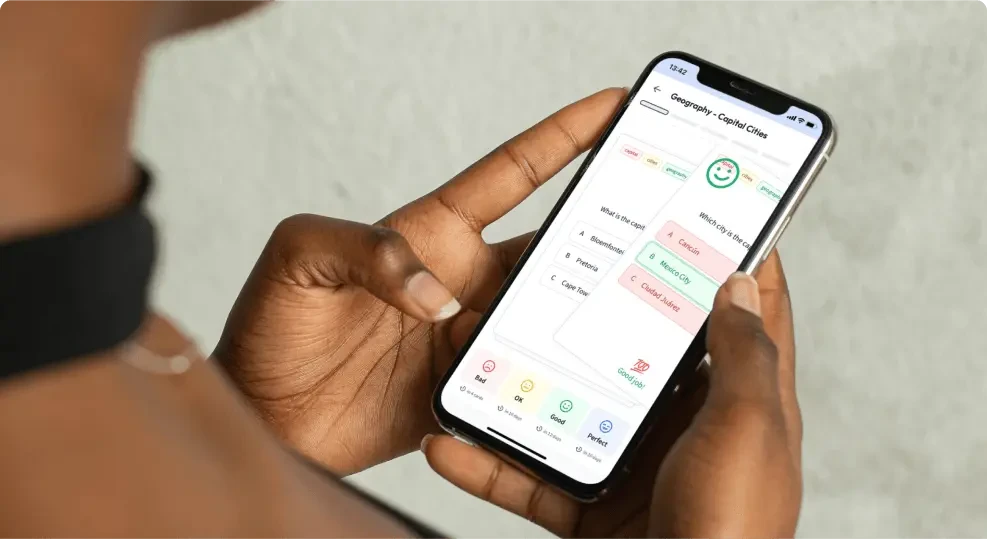
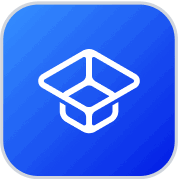
About StudySmarter
StudySmarter is a globally recognized educational technology company, offering a holistic learning platform designed for students of all ages and educational levels. Our platform provides learning support for a wide range of subjects, including STEM, Social Sciences, and Languages and also helps students to successfully master various tests and exams worldwide, such as GCSE, A Level, SAT, ACT, Abitur, and more. We offer an extensive library of learning materials, including interactive flashcards, comprehensive textbook solutions, and detailed explanations. The cutting-edge technology and tools we provide help students create their own learning materials. StudySmarter’s content is not only expert-verified but also regularly updated to ensure accuracy and relevance.
Learn more