Jump to a key chapter
Understanding Magnetization
Magnetization, an intriguing phenomenon in Physics, is linked to fascinating applications in everyday life. Uncovering the sense of magnetization helps unravel the mysteries of mysterious magnetic fields and their consequent physical properties.Definition of Magnetization: Unveiling the Basics
Let's embark on the journey to apprehend magnetization.Magnetization, denoted by \(M\), refers to the measure of the magnetic moment per unit volume of a material. In simplistic terminology, it is the degree to which a material gets magnetized when exposed to an external magnetic field.
Core Concept of Magnetization
Appreciating the core concept of magnetization demands understanding the behaviour of magnetic dipoles under an applied magnetic field. Every atom in a material has an intrinsic magnetism due to the orbital and spin movements of its electrons. When a magnetic field \(B\) is applied, these atomic dipoles tend to line up with the direction of \(B\) contributing to the net magnetization of the material.For instance, imagine a class being the material and the students being atomic magnetic dipoles. A strict teacher, representing the external magnetic field, enters, causing the chattering students (random atomic dipoles) to become quiet and attentive, aligning in the teacher's desired manner (direction of the external field). This simple analogy helps visualize how magnetization works on the atomic level.
Importance of Magnetization in Physics
Perceiving magnetization's significance is essential to understand its vast applications and phenomenon in physics.- Magnetization underpins the understanding of electromagnetism, where it contributes to the overall magnetic field alongside the applied field.
- The concept plays a crucial role in technologies like MRI scanners, wherein the magnetization of atomic nuclei is crucial for image creation.
- Magnetization also contributes to information storage, as in hard disks, where each bit of data is stored as the magnetization direction in a small magnetic domain.
An interesting aspect of magnetization is the phenomenon of hysteresis. When a magnetic field is applied and then removed, some materials retain their magnetization, exhibiting a 'magnetic memory'. This characteristic is harnessed in devices like magnetic tapes and hard drives for data storage.
Exploring the Magnetic Field
The magnetic field, a fundamental component of magnetization, is an unseen force field that exists due to moving electric charges or inherent magnetic properties in certain materials. It's essential to discern the magnetic field’s role to comprehend magnetization thoroughly.Interaction of Magnetic Field with Magnetization
When considering how a magnetic field interacts with magnetization, imagine the magnetic field as a 'magnetic wind', influencing the alignment of the atomic magnetic 'weathervanes' within a material. Picture it at both macroscopic and atomic levels. On a larger scale, the applied magnetic field, denoted as \(H\), forces the material’s magnetic polarity to align with its direction. On the atomic level, the tiny magnetic dipoles within the material attempt to align themselves with this applied field. The combined tendency towards this alignment results in magnetization of the material. An intriguing concept to explore here is magnetic susceptibility (\(\chi\)) which sheds light upon the interaction between the magnetic field and magnetization. Magnetic susceptibility is a proportionality constant that indicates how much magnetization a material gains in an external magnetic field. The higher the material's susceptibility, the greater its magnetization in a given magnetic field. Conversely, a material with low susceptibility will gain little magnetization even under a strong magnetic field. Clearly, this interaction is fundamental in our understanding of magnetic phenomena. But what role does this magnetic field play in magnetization?Role of Magnetic Field in Magnetization
The magnetic field serves as the external influencing parameter that incites or initiates the process of magnetization. Essentially, it acts as a driving force to align the atomic dipoles within a material to result in a net magnetization. Its role is embodied in the simple yet remarkable equation: where \(M\) represents the magnetization, \(\chi\) is the magnetic susceptibility, and \(H\) is the strength of the applied magnetic field. As evident from the equation, the magnetic field directly influences the magnetization for a given susceptibility.Effect of Magnetic Field Strength on Magnetization
The applied magnetic field strength has a significant influence on the degree of magnetization. A stronger magnetic field induces greater alignment of magnetic dipoles, thus, increasing the material's magnetization. However, this relationship isn't linear. Upon reaching a certain threshold known as saturation magnetization, increasing the magnetic field strength doesn't raise the magnetization further. Let's summarise this interaction in a table:Magnetic Field Strength (H) | Effect on Magnetization (M) |
Low | Low magnetization, few atomic dipoles aligned |
Increasing | Increase in magnetization as more dipoles align |
Saturation Point | Maximum magnetization, all dipoles aligned |
Beyond Saturation Point | No increase in magnetization |
Magnetization Techniques for Learning
Physics, like magnetization, is a practical oriented subject. It's not just about mastering the theoretical concepts, equations or definitions. To truly understand magnetization, you need hands-on experience with magnetization techniques. This will deepen your understanding and make learning more enjoyable.Basic Techniques for Magnetization
Mastering the concept of magnetization requires a good grounding in both theory and practice. Here are some fundamental techniques to help you grasp magnetization.The first technique is direct current magnetization (DC magnetization), which carries out magnetization through the use of a steady current. Materials are magnetized by allowing a direct current to flow through a coil wound around the material.
- Carry out experiments in a laboratory setup
- Comprehend the mathematical expressions that govern these techniques
- Review practical applications and phenomena to see these techniques in action
Theory Behind Magnetization Technique
Diving into the physics behind magnetization techniques, each of these methods is based on the principle of aligning the atomic magnetic dipoles within a material using an external magnetic field.In DC magnetization, a steady or time-invariant external field aligns the magnetic dipoles. The magnetization \(M\) of the material increases in proportion to the applied field \(H\) as given by \(M = \chi H\), until saturation is reached, beyond which it remains constant.
Practical Examples of Magnetization Technique
Each magnetization technique finds various practical applications and classroom demonstrations. Using a simple laboratory setup with a power source and a solenoid coil, you can demonstrate DC magnetization. By connecting a ferromagnetic material like iron to the DC power source via a coil, you should observe that the material becomes magnetized and can attract other magnetic objects. To illustrate AC magnetization, one could use an AC source instead of a DC one. The magnetic behaviour of the material changes with AC; instead of staying magnetized, you see it vibrating due to the change in magnetic field direction with alternating current. A notable application of pulsed field magnetization is the magnetization of superconducting materials. These materials exhibit zero electrical resistance and perfect diamagnetism (complete expulsion of magnetic fields) at very low temperatures (below their critical temperature). Because normal magnetizing fields aren't strong enough to penetrate the superconducting state, pulsed fields capable of momentarily overwhelming the superconductor's diamagnetism are used to impress the desired magnetization. Understanding the theory and practical examples of these magnetization techniques will undoubtedly enrich your understanding of the physics of magnetization.Deep Dive into the Meaning of Magnetization
The term "Magnetization", a key concept in physics, essentially refers to the process by which certain materials respond to an applied magnetic field. To understand magnetization in its entirety, it's crucial to delve into the historical background, its modern definition, and the inherent relationship it has with the magnetic field.Historical Background of Magnetization Meaning
Magnetization's roots trace back to the ancient Greeks' discovery of the naturally occurring lodestone, a naturally magnetized form of the mineral magnetite. They noticed that these unique stones could attract iron pieces and other lodestones. Over time, the concept of magnetism developed, leading to the term "magnetization". The 18th and 19th centuries saw significant advancements in the understanding of magnetization. It was during this period that magnetization was formally defined and studied. It was Andre-Marie Ampere who suggested that all magnetism is the result of countless tiny magnetic dipoles aligned together. Hannes Alfvén further refined the concept, proposing the magnetohydrodynamics theory that illustrates magnetization’s role in plasmas. To discuss the process of magnetization, a critical early concept is retentivity or remanence. This is a measure of a magnet's ability to remain magnetized after removal of the inducing field. Also, coercivity, the magnetic field strength required to demagnetize a magnet. The history of magnetization demonstrates the evolution of understanding towards this fundamental physical concept.Modern Interpretation of Magnetization Meaning
In contemporary physics, magnetization refers to the measure of the magnetic moment per unit volume of a material. It is representative of the degree of alignment of atomic magnetic dipoles within the material. The modern interpretation of magnetization hinges on the concept of atomic magnetic dipoles. These are minute magnets within a material that align under the influence of an external magnetic field. The overall magnetization of a material, symbolised by \(M\), depends on the extent of this dipole alignment. The formula that encapsulates the modern understanding of magnetization is: where \(\chi\) is the susceptibility indicating the ease with which a substance can be magnetized, and \(H\) is the applied magnetic field. When a magnetic material is exposed to an external magnetic field, a remarkable transformation occurs. The random state of atomic dipoles in the material shifts towards aligning with the external field, thereby inducing magnetization.Relation between Magnetization Meaning and Magnetic Field
The inherent relationship between magnetization and the magnetic field is fundamentally crucial to understanding magnetization. Essentially, the magnetic field is the external stimulus that incites magnetization by aligning the atomic dipoles. This indispensable relationship is made precise by the magnetization equation: The equation illustrates that for a specific material (with given susceptibility, \(\chi\)), the magnitude of the magnetization is directly proportional to the applied magnetic field \(H\). The more potent the external magnetic field, the more substantial the magnetization. This interactive effect of the magnetic field and magnetization is also instrumental in explaining the concept of magnetic hysteresis. This describes a lagging effect where the magnetization of a material does not immediately follow changes in the magnetic field, resulting in a unique hysteresis loop when magnetization is plotted against the magnetic field strength. Thereby, the interplay between magnetization and the magnetic field represents a fundamental cornerstone in the realm of magnetic phenomena. Understanding this core relation is the key to unlocking the intricate world of magnetization in physics.Causes of Magnetization: A Comprehensive Guide
Magnetization, the process by which certain materials acquire and exhibit magnetic properties, doesn't happen spontaneously. It is influenced and mediated by several factors, from the external environment to the inherent physical properties of the material involved. To better understand the underlying causes of magnetization, we delve into the primary factors and their roles in this fascinating physical process.Primary Factors Leading to Magnetization
Primarily, the causes of magnetization can be distilled into two broad categories: external influences such as magnetic fields and temperature, and inherent physical properties of the material such as atomic structure and magnetic domains. Each of these factors plays a critical role in determining whether, how and to what extent a material gets magnetized.The external magnetic field, represented as \(H\), is a significant driver of magnetization. When a material is subjected to an external magnetic field, its atomic dipoles - tiny atomic magnets - align with the field. The degree of alignment, and thus the resulting magnetization, is mediated by the strength of the applied magnetic field. Mathematical representation of this concept is \(M = \chi H\), where \(M\) is magnetization and \(\chi\) is the material's magnetic susceptibility.
Environmental Influences on Magnetization Causes
Beyond magnetic field and temperature, the environment itself can also substantially influence a material's magnetization. For instance, pressure and mechanical stress can affect the alignment of atomic dipoles. Increased pressure can result in greater dipole alignment, resulting in an intensified magnetization. Radiation can also cause magnetization in certain materials. This is due to the phenomenon of spintronics, where electron spins (which constitute atomic dipoles) can be manipulated using light, leading to a change in the material's magnetization state.Role of Physical Properties in Magnetization Causes
The role that innate material properties play in magnetization is just as essential. Here, the atomic structure and magnetic domains are the key players in determining the extent and character of a material's magnetization. The atomic structure affects magnetization due to the presence of unpaired electrons in an atom's outer shell. These unpaired electrons possess an intrinsic magnetic moment contributing towards the magnetic properties of an atom.For instance, iron is highly magnetic due to the presence of four unpaired electrons in its outermost shell. This results in a high atomic magnetic moment, causing iron to be readily magnetizable
Magnetization - Key takeaways
- Magnetization is a fundamental aspect of electromagnetism, it contributes to the overall magnetic field alongside the applied field, and is fundamental in technologies like MRI scanners and information storage.
- Magnetization contributes to hysteresis, a property where certain materials retain their magnetization even when an external magnetic field is removed, creating a 'magnetic memory' which is utilized for data storage in devices like hard drives.
- The magnetic field, a key component of magnetization, exists due to moving electric charges or inherent magnetic properties in materials, influencing the alignment of the atomic magnetic 'weathervanes' within a material, leading to its magnetization.
- Magnetic susceptibility is a measure of how much a material will become magnetized in an external magnetic field. It impacts the interaction of a magnetic field with magnetization.
- The greater the applied magnetic field strength, the greater the degree of magnetization in a material, as a stronger magnetic field induces greater alignment of magnetic dipoles. However, beyond a certain saturation point, increasing the magnetic field strength does not further increase the magnetization.
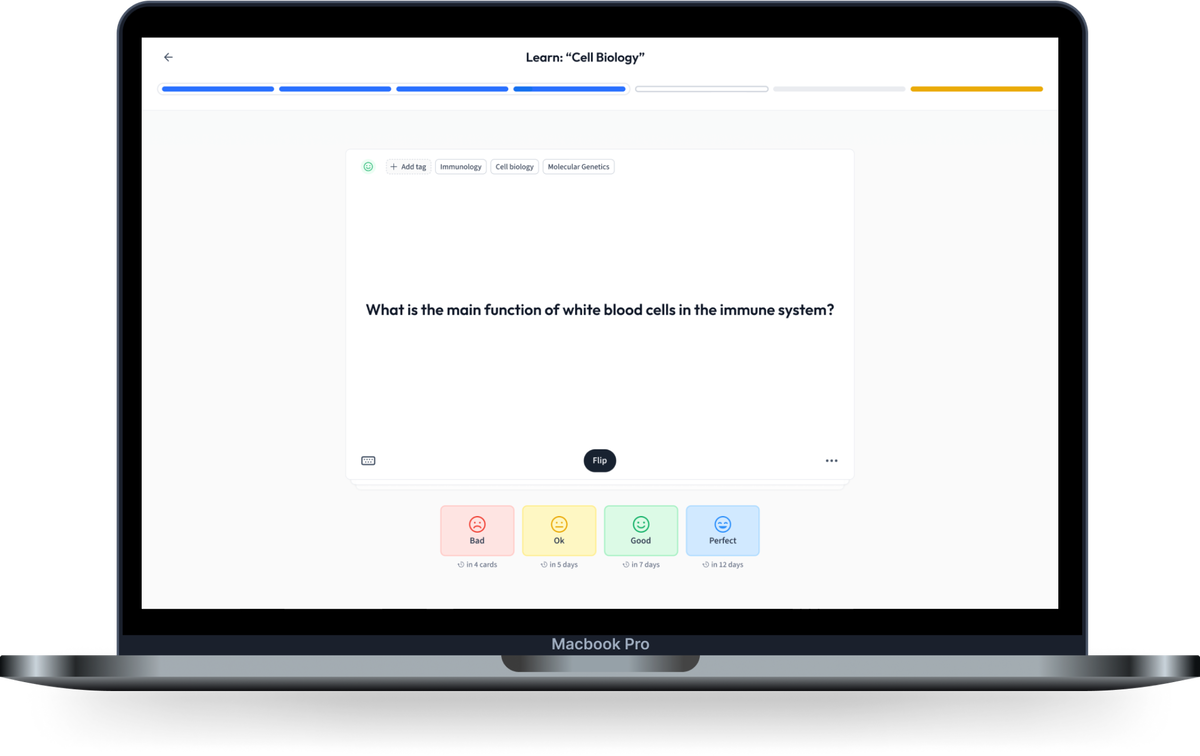
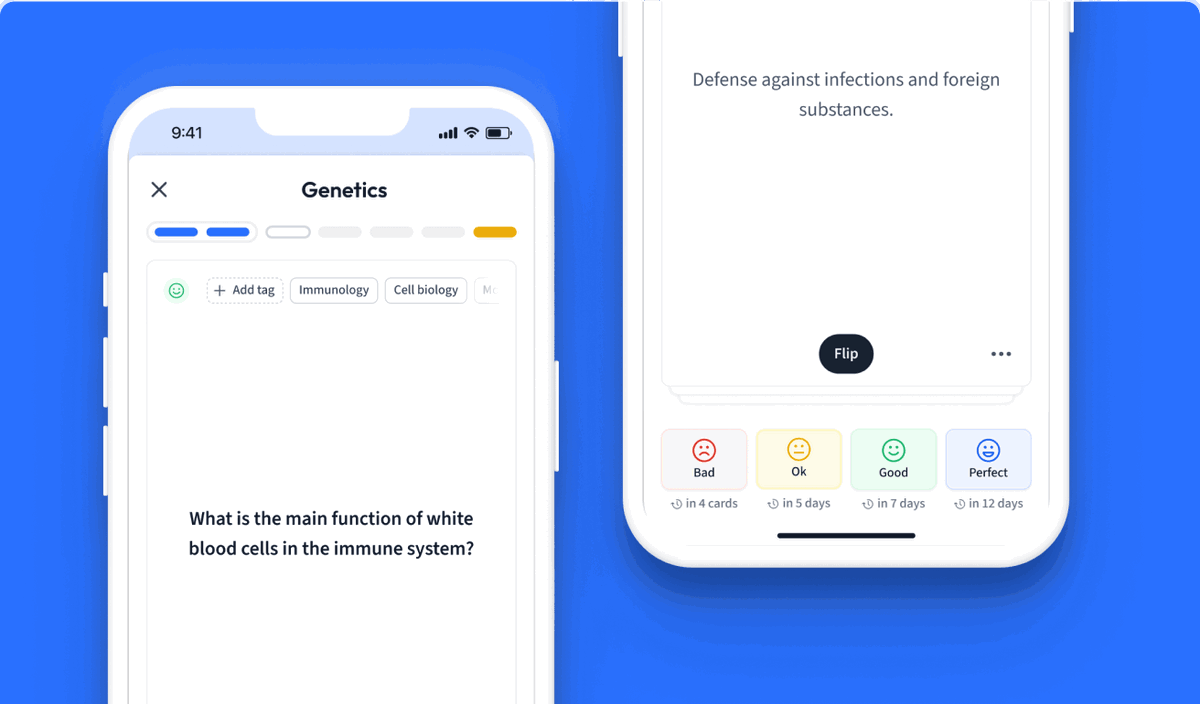
Learn with 15 Magnetization flashcards in the free StudySmarter app
We have 14,000 flashcards about Dynamic Landscapes.
Already have an account? Log in
Frequently Asked Questions about Magnetization
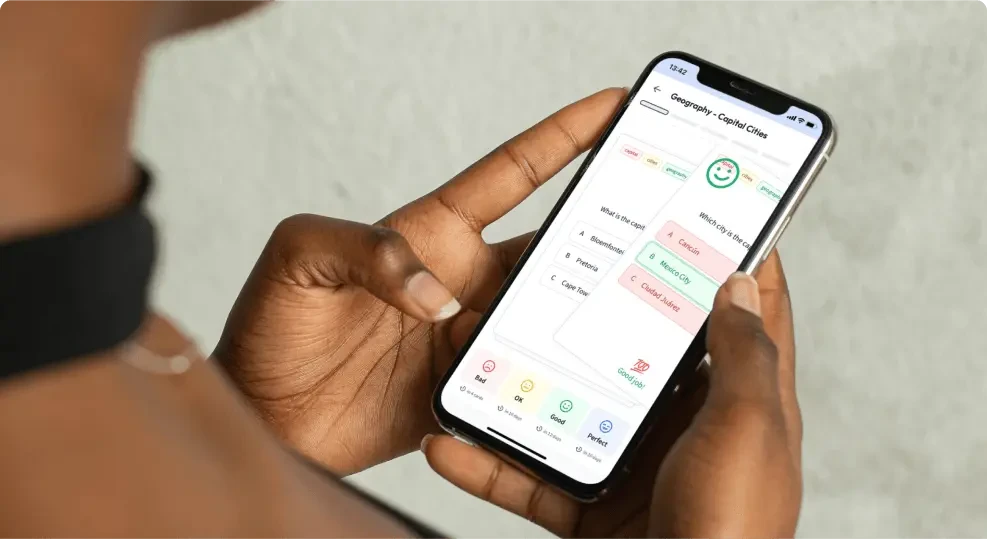
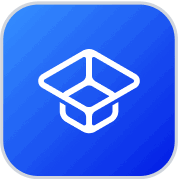
About StudySmarter
StudySmarter is a globally recognized educational technology company, offering a holistic learning platform designed for students of all ages and educational levels. Our platform provides learning support for a wide range of subjects, including STEM, Social Sciences, and Languages and also helps students to successfully master various tests and exams worldwide, such as GCSE, A Level, SAT, ACT, Abitur, and more. We offer an extensive library of learning materials, including interactive flashcards, comprehensive textbook solutions, and detailed explanations. The cutting-edge technology and tools we provide help students create their own learning materials. StudySmarter’s content is not only expert-verified but also regularly updated to ensure accuracy and relevance.
Learn more