Jump to a key chapter
Understanding the PN Junction
The PN Junction is a cornerstone topic in the study of physics, particularly in the exploration of semiconductors and the glamorous world of electronics. It serves as a fundamental component in numerous electronic devices that shape your everyday life.What is a PN Junction: Definition and Importance
A PN Junction refers to the boundary or interface between two types of semiconductor material, 'p' and 'n' typed, in a single crystal of semiconductor.
Imagine a border between two countries, one country representing the P-type material and the other, the N-type material. The border or boundary between these two represents the PN Junction, controlling the flow of citizens (current) between the two.
Did you know that the first PN junction semiconductor was a copper oxide rectifier, invented by Tetsuro Uchikawa in 1931 at the Research Institute of Electrical Communication of Tohoku University, Japan? Over the years, this technology has incredibly evolved and is widely utilised in everyday electronics.
The Formation Process of a PN Junction
The PN Junction forms right where a N-type semiconductor meets a P-type. To start with, think of a pure silicon crystal, known as intrinsic silicon. It is neither P-type nor N-type. But this changes dramatically as we introduce doping. Using different doping elements, part of this crystal becomes a P-type and the rest, an N-type. Here is a step by step procedure of how this happens:- \( P \)-type Semiconductor: Introduce a trivalent atom (like boron) into the silicon crystal. Each of these boron atoms will release a hole, making silicon a positive carrier or 'p' type.
- \( N \)-type Semiconductor: Introduce a pentavalent atom (like phosphorus) into the silicon crystal. Each of these phosphorus atoms releases an extra electron, making silicon a negative carrier or 'n' type.
To grasp the process, consider an ice cream parlor. An intrinsic silicon is like a big scoop of plain vanilla ice-cream. But when we introduce the 'doping' elements, akin to various yummy toppings and sauces, it transforms. The vanilla scoop infused with caramel sauce (i.e., boron) becomes the delectable caramel-flavored \( P \)-type. On the other hand, the same scoop blended with choco chips (i.e., phosphorus) becomes the irresistible choco-chip flavored \( N \)-type. When these two half-and-half scoops are combined, the interface is the PN Junction!
This very characteristic of controlling the direction of current flow makes PN Junction the fundamental principle behind many electronic devices.
Exploring the Characteristics of a PN Junction
Diving further into the fascinating world of semiconductors, it's critical to comprehend the unique characteristics of a PN Junction. These characteristics, irrespective of the device or context, remain constant and crucial to the functioning of electronic devices.An Overview of PN Junction Theory
The high-level theory of a PN Junction circulates around the ideas of its depletion region, forward bias, reverse bias, and the resultant rectifying property.
- Forward bias: When the P-type is connected to the positive of the power supply and the N-type to the negative, it's forward bias. In this condition, the depletion region narrows, and current flows across the junction.
- Reverse bias: When the P-type is connected to the negative of the power supply and the N-type to the positive, it's reverse bias. In this state, the depletion region widens and, technically, no current should flow since the negative terminal repels electrons and the positive terminal repels holes. However, due to minute thermal ionisation, a tiny leakage current flows. This current is named reverse saturation current.
Imagine a one-way gate at a theme park. In one scenario, you're permitted to go through (like forward bias, where current flows). In the other, the gate is closed, and you're not allowed to move forward (like reverse bias, where ideally no current flows).
PN Junction Diode: Essential Understanding
The simplest application of a PN Junction is a PN Junction diode. When it comes to practical electronics, remembering the fundamental theorem, "\(\textit{In a Diode, forward bias leads to current, while reverse bias doesn't,}" is vital.A PN Junction Diode is a device that operates under the principle of a PN Junction. With the forward and reverse bias characteristics, the diode controls the electric current flow.
Bias | Current flow? |
Forward Bias | Yes |
Reverse Bias | No (Except reverse saturation current) |
A diode is like a traffic cop, diligently ensuring that current only travels in the direction it's allowed to. In a traffic scenario, this could be a one-way street, and the traffic cop (diode) ensures traffic flows only in the permitted direction.
Delving into the Regions of PN Junction
When discussing the breadth and depth of a PN Junction's characteristics, it is quintessential to familiarise yourself with its regions. Specifically, the Depletion Region warrants particular attention due to the crucial role it plays in governing a PN Junction's performance.Depletion Region in PN Junction: A Detailed Discussion
In the wider scheme of a PN Junction, the Depletion Region constitutes a pivotal element and displays several fascinating characteristics. By name, you might imagine it as a realm of nothingness, but in reality, it's an area bustling with potential differences, electric fields, and insightful principles.The Depletion Region in a PN Junction refers to a zone where mobile charge carriers, namely holes and electrons, are mostly absent. This depletion of charge carriers is the reason for its naming.
The potential difference across the Depletion Region of a PN Junction, often referred to as Junction Voltage or Built-In Potential, implicates the force needed to move a unit positive charge from the P-type to the N-type semiconductor against the electric field, or equivalently, the energy required to move an electron in the opposite direction.
The Function and Role of Depletion Region
At the heart of a PN Junction's unique qualities, the Depletion Region channels an array of pivotal functions that drive the quintessential mechanisms and behaviour that make devices such as diodes and transistors feasible. Foremost, the Depletion Region hosts the electric field, which emerges due to the diffusion of majority carriers across the junction. This electric field lays the foundation for a significant principle in electronics: it allows the current to flow in one direction and restricts it in another. The field propels the free electrons in the N-type region towards the negative terminal of the power supply and does the same with the holes in the P-type region towards the positive terminal. As a result, a PN junction only conducts when it is forward-biased—this characteristic is known as unidirectional conductance. Moreover, the thickness of the Depletion Region dramatically shifts depending upon whether the PN Junction is forward or reverse biased. Under forward bias, the applied voltage opposes the built-in potential, causing the Depletion Region to shrink and enabling carriers to cross the junction. On the other hand, with reverse bias, the applied voltage strengthens the built-in potential, widening the Depletion Region, and therein inhibiting the flow of carriers. Thus, the Depletion Region stands as a metaphorical 'gate-keeper,' mediating and monitoring the flow of carriers, which translates to the current in a circuit.The Depletion Region is akin to the management system controlling the flow of visitors in a large amusement park. Depending on different conditions (like a special event or safety concerns), this system might open several gates (resembling Forward Bias), allowing many visitors to stream in, or close most of them (corresponding to Reverse Bias), permitting only a few to enter.
Understanding Forward and Reverse Bias in PN Junction
The principles of forward and reverse bias stand at the core of a PN Junction's operation. Fully comprehending these concepts opens up the intrigue and elegance behind how a myriad of electronic devices function.Forward Bias PN Junction: How it Works
An understanding of a forward bias PN Junction necessitates revisiting the essential constituents of a PN Junction - the P-type and N-type semiconductors, and appreciating the role of an external voltage source.In a forward bias setup, the positive terminal of a voltage source is connected to the P-type semiconductor, and the negative terminal is connected to the N-type semiconductor.
Reverse Bias PN Junction: Its Role and Function
Contrasting to the forward bias, a reverse bias PN Junction involves connecting the negative terminal of the external voltage supply to the P-type region, and the positive terminal to the N-type region.A reverse bias is a scenario where an external voltage is applied such that it enhances the electric field across the PN junction, effectively widening the depletion region and reinforcing the barrier that prevents the majority charge carriers from traversing across the junction.
Applying Knowledge of PN Junction
Turning theoretical understanding into practice widens the horizon of any scientific concept. So, when you unlock the intricacies of a PN Junction, you unravel a vast realm of practical possibilities.Practical Applications of PN Junction Diode
The PN Junction Diode, a simple yet indispensable electronic device, excels in various applications — right from the primary tasks in low-power electronics to the sophisticated strides in advanced communication systems. The key to the PN Junction Diode's widespread applicability lies in its fundamental principle— it exhibits unidirectional conductance. Due to this property, it conducts current in one direction (forward bias), while blocking it from the other (reverse bias). An array of practical applications spring from this rudimentary behaviour:- Rectification: The most common application of a diode is in power supply circuits to convert Alternating Current (AC) into Direct Current (DC). This process is known as rectification. The diode allows current to pass only during one half of the AC cycle, effectively 'rectifying' the AC into a unidirectional current flow, reminiscent of DC.
- Clipping and Clamping Circuits: Diodes also find utility in signal processing circuits, specifically clipper and clamper circuits. Clipping circuits are utilised to 'clip' off a portion of a signal above or below certain DC levels, while clamping circuits are designed to shift the entire signal to a desired DC level.
- Voltage Multiplier Circuits: In high voltage applications, diodes can aid in acquiring voltages higher than the input peak voltage without the necessity of a transformer, a role served by voltage multiplier circuits.
- Protection Circuits: Diodes are widely used in protection circuits. For example, Zener diodes can be used as voltage regulators in circuits, protecting other components against voltage surges.
- Switching Applications: In digital logic circuits and computer systems, diodes are often used as switches controlling current flow in various parts of the circuit.
Case Studies: Effective Use of PN Junction in Real-world Scenarios
Real-world applications and case studies lend weight to the theoretical concepts of PN Junction. Through the lens of tangible, practical scenarios, the versatility and impact of this fundamental semiconductor structure magnifies dramatically. Consider the world of telecommunications, wherein the PN junction diode — specifically, the Zener diode — plays a crucial role. Zener diodes act as voltage regulators, ensuring a stable, constant voltage supply, thereby protecting the intricate electronic equipment used in telecommunication networks from voltage surges. The influence of the humble PN Junction extends towards the realm of biomedical research, too — particularly, the inferred temperature sensors. These PN junction devices operate under the principle that the voltage across the diode changes with temperature. By precisely calibrating these devices, scientists and engineers can use them for accurate temperature measurements in sensitive and subtle biological studies. On another front, the domain of automobile electronics leans on the PN Junction for vital tasks like alternator voltage regulation. In vehicles, alternators generate an Alternating Current (AC) that needs to be 'rectified' into a Direct Current (DC) for charging car batteries and powering its electrical system. This rectification is possible due to the PN Junction diode, which ensures the smooth and efficient running of vehicles' electrical sub-systems. Moreover, visionaries in the field of clean energy have incorporated the principles of PN Junction in harnessing solar power. Solar, or photovoltaic, cells work on the very fabric of PN Junction principles. When solar energy impinges on the PN Junction in these cells, it generates electron-hole pairs, which under the influence of the internal electric field of the junction, contributes to the current in the circuit. In each of these instances, the behind-the-scenes hero is our simple yet versatile PN Junction, underpinning various facets of technological progress. Ultimately, knowledge of PN Junction and its principles can open doors to numerous practical applications and innovations.PN Junction - Key takeaways
- PN Junction is fundamental to many electronic devices and controls the direction of current flow. It arises due to the movement of free electrons (N-type) towards holes (P-type), creating a voltage equilibrium.
- The essential concepts in PN Junction theory include depletion region, forward bias, reverse bias, and rectifying property. Depletion region is where no mobile charge carriers (holes and electrons) are present.
- In forward bias, P-type is connected to the positive of the power supply and N-type to the negative, the depletion region narrows, and current flows. In reverse bias, P-type is connected to the negative of the power supply and N-type to the positive, the depletion region widens, and very limited current flow occurs due to minute thermal ionisation.
- A PN Junction diode is the simplest application of a PN Junction, operating under the principle of forward and reverse bias. Forward bias leads to current, while reverse bias does not, except for a minute reverse saturation current.
- The depletion region in a PN Junction plays a crucial role as it depletes charge carriers, forms an electric field, and establishes a potential difference, often referred to as junction voltage or built-in potential. This voltage can be calculated as \( V_j = \frac{{kT}}{{q}} \ln\left(\frac{{N_a N_d}}{{n_i^2}}\right) \).
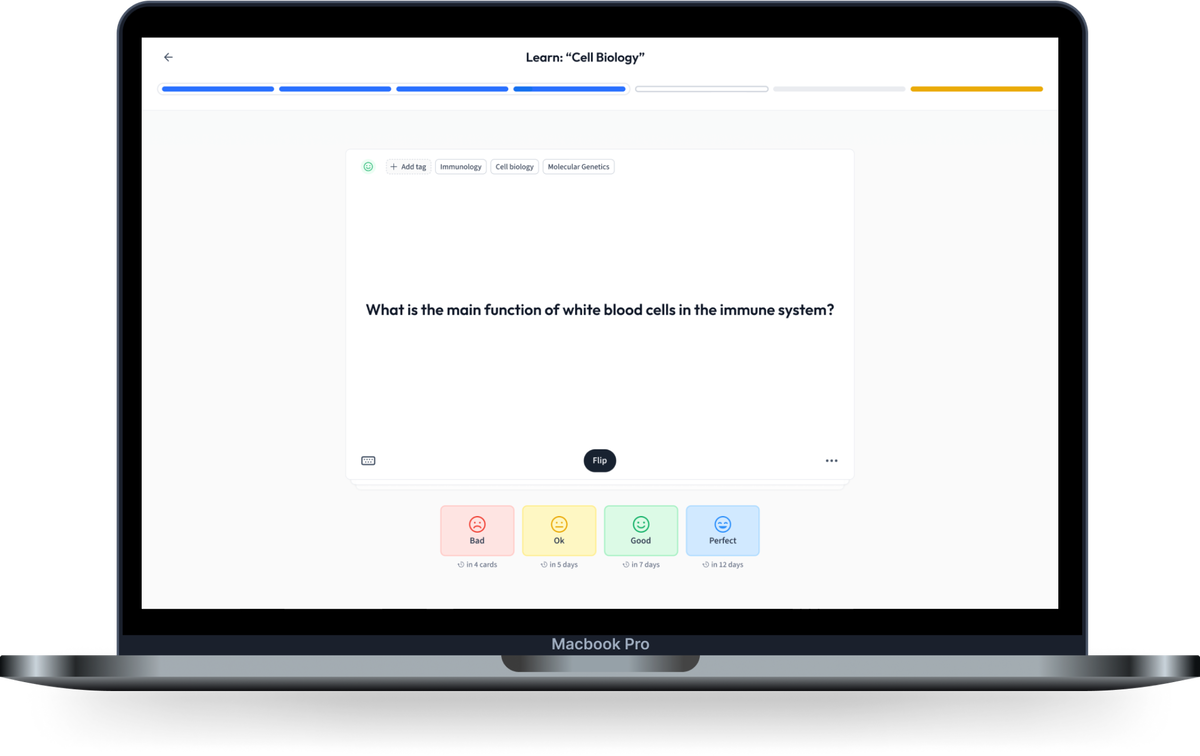
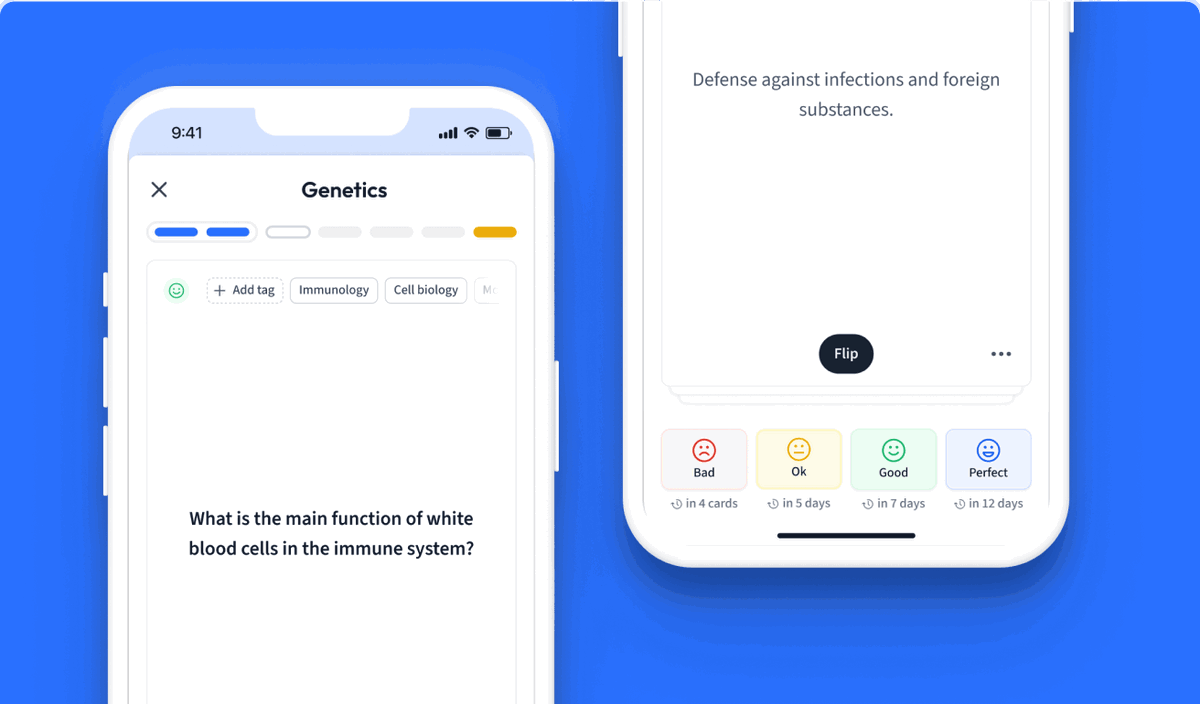
Learn with 15 PN Junction flashcards in the free StudySmarter app
We have 14,000 flashcards about Dynamic Landscapes.
Already have an account? Log in
Frequently Asked Questions about PN Junction
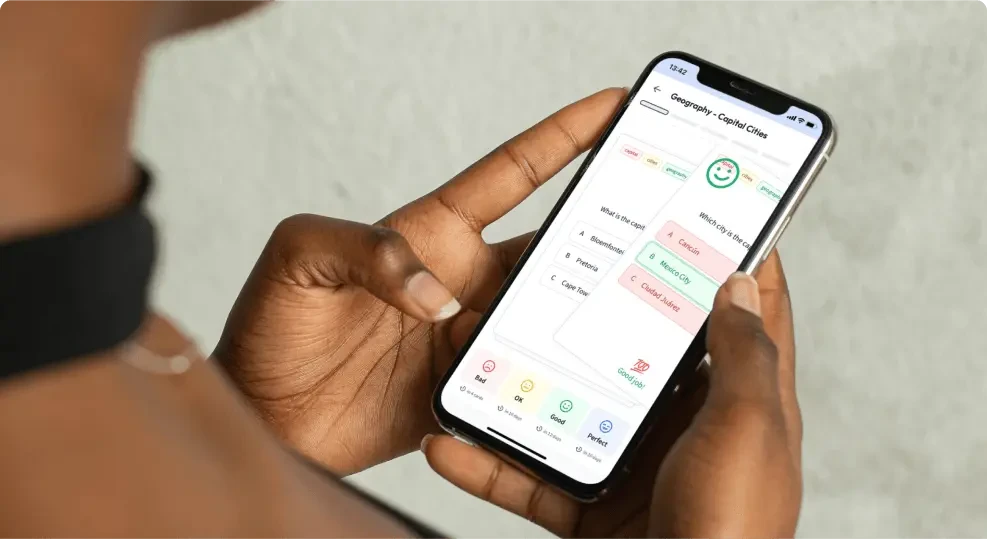
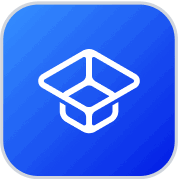
About StudySmarter
StudySmarter is a globally recognized educational technology company, offering a holistic learning platform designed for students of all ages and educational levels. Our platform provides learning support for a wide range of subjects, including STEM, Social Sciences, and Languages and also helps students to successfully master various tests and exams worldwide, such as GCSE, A Level, SAT, ACT, Abitur, and more. We offer an extensive library of learning materials, including interactive flashcards, comprehensive textbook solutions, and detailed explanations. The cutting-edge technology and tools we provide help students create their own learning materials. StudySmarter’s content is not only expert-verified but also regularly updated to ensure accuracy and relevance.
Learn more