Jump to a key chapter
Understanding Transduction in Modern Physics
The concept of transduction plays a crucial role in modern physics. It is a broad subject that imbues a multitude of physical phenomena, from the smallest quantum interaction to the largest cosmological event. Transduction is omnipresent in everyday life, though often unnoticed, yet it's the heart of countless technologies you use daily.The Basic Concept: What is Transduction?
Transduction, in the context of physics, is the process of converting one form of energy into another. This conversion can happen in various ways and is defined by the interaction between distinct physical systems or within one system.
- Thermal to electrical energy: Thermo-electric generator
- Light to electrical energy: Solar panels
- Mechanical to electrical energy: Dynamo
Historical Background of Transduction in Physics
The history of transduction is tightly intertwined with the development of physics and our understanding of energy. Predating our comprehension of energy, our ancestors used forms of transduction intuitively in their daily lives.A primitive form of transduction is the use of a simple lever. The lever converts the energy of our muscles into a higher force that allows lifting heavy objects – a mechanical to mechanical energy transduction.
The understanding of transduction in physics took a giant leap in the 19th century with the formulation of the laws of thermodynamics. These laws expressed the rules governing energy conversion and laid the foundation for understanding transduction mechanisms in the modern sense.
Period | Transduction Milestone |
Ancient Times | Simple levers and mechanical devices |
19th Century | Formulation of the laws of thermodynamics |
20th Century | Development of electrical and electronic transducers |
21st Century | Advancements in nanoscale and wireless energy conversions |
Delving into Transduction Process in Physics
In Physics, the all-encompassing discipline that studies the universe and its natural phenomena, the concept of transduction holds a paramount position. Transduction, put simply, is the process of converting one form of energy into another. It's like a magician's hat, albeit one governed by scientifically established laws, from which a rabbit (energy in one form) goes in and a dove (energy in another form) emerges.Typical Examples of Transduction in Physics
Several practical realisations of transduction exist around us. Each one highlights a unique transformation. Here are some typical examples:- Microphone: A microphone converts sound energy (acoustic energy) into electrical energy. The energy of the sound waves makes a diaphragm in the microphone vibrate. This vibration then causes an electrical signal of corresponding frequency to be produced.
- Loudspeaker: The loudspeaker is a transducer that works in a manner just opposite to the microphone. Electrical energy feeds into the speaker as an amplified signal, which causes a diaphragm to vibrate. This vibration generates sound waves in air, converting electrical energy into acoustic energy.
- Solar Panel: A solar panel is another common example of transduction. It converts sunlight (a form of electromagnetic radiation) into electrical energy. This conversion happens courtesy of photovoltaic cells in the solar panel, which generate an electrical voltage when light falls on them.
Key Steps in a Physics Transduction Process
While the specific mechanism varies with different types of transducers, certain basic principles remain consistent. Accordingly, the key steps include: 1. Input Energy: The transduction process starts by feeding an input energy into the transducer. This energy can be of any form— mechanical, electrical, electromagnetic, thermal, or chemical. 2. Energy Conversion: Once the transducer receives the input energy, it begins the conversion process. Depending on the type of transducer, this might involve mechanical movement (as in a microphone), excitation of electrons (as in a solar panel), or other physical phenomena. 3. Output Energy: The transducer then generates output energy, a different form from the input energy. For instance, a dynamo converts mechanical energy (expressed as rotational motion) into electrical energy. 4. Performance and Efficiency: This step deals with assessing the efficiency of the transduction process. Not all input energy effectively transforms into the desired output energy— some dissipates as waste, typically heat. Efficiency (\( \eta \)) can be calculated using the formula: \[ \eta = \left(\frac{\text{useful energy output}}{\text{total energy input}}\right) \times 100\% \]How Physics Transduction Works: A Detailed Look
To understand the transduction process in-depth, we'll break it down into constituent parts. First, consider a transduction event as an energy exchange happening within a defined system. This system, the transducer, is an interface that connects two energy domains— input and output. What happens to the energy within this system follows the fundamental laws of physics. Let's delve deeper into the dynamics of this energy exchange.The Input and Output Concept of Transduction
In any transduction process, energy in one form— the input— enters the transducer. Here, the energy undergoes a transformation involving physical mechanisms specific to the type of transducer. This transformation results in energy of another type— the output— leaving the transducer. Consider the example of a hydroelectric plant. Here, the gravitational potential energy of water (input) transforms into mechanical energy as the water rushes down and turns a turbine. The turbine's rotational mechanical energy then transforms into electrical energy through an electric dynamo. Despite similarities in basic operating principles, each transducer has unique specifics. An acoustic transducer, like a microphone, deals with mechanical vibrations triggered by sound waves, while a photovoltaic cell is concerned with the absorption and absorption of photons from sunlight. No matter the specifics, all types of transduction honour the supreme dictum of physics: Energy cannot be created nor destroyed— only transformed. This inviolable rule governs the transduction process, ensuring energy conserves as it morphs from one form into another within the magical hat of the transducer.Decoding Principles of Transduction in Physics
The concept of transduction might seem deceptively simple at first glance. However, the fundamental principles that govern this grand act of energy transformation run deep, unveiling the intricate interplay of the universe’s elements. Understanding these principles offers a profound insight into the mechanics of energy exchange and the rules underpinning our physical realities.Core Transduction Principles in Physics
The principles behind the process of transduction focus on energy's continuous dynamic dance from one form to another. The overarching guidelines governing this dance include the laws of conservation and dissipation of energy and principles related to transducer function. To gain a comprehensive look, a meticulous understanding of these principles is crucial.Laws of energy conservation and dissipation: At the heart of energy transduction lies the principle of energy conservation. According to this law, while energy may transform in form, its total quantity in a closed system remains constant. Expressed mathematically, majority of transduction processes follow this equation: \[ \text{Input Energy} = \text{Output Energy} + \text{Energy Loss} \]
- Resistance: Physical systems are subject to internal and external resistances that impede energy transfer, resulting in energy loss as heat.
- Impedance Mismatch: When two energy systems fail to align their impedance levels properly during an energy transfer, some energy reflects back, resulting in an energy loss.
Principles related to transducer function: Transducers, which form the core of transduction, operate based on diverse principles, with each transducer type having its unique conversion mechanism.
How Transduction Principles Influence Physics Theories
The principles of transduction not only form the crux of energy transformation but also aid in sculpting several theories and fields in physics. An insightful observation of these principles refines our understanding of energy's functionality, guides further research, and propels technical innovation. For example, the 'principle of conservation of energy' is a universal principle that interlinks various physical theories. From establishing the fundamentals of mechanics to orchestrating quantum mechanics, this principle is instrumental in composing unified theories of physics. Transducers function on a variety of physics theories, and advancements in their understanding or technology often lead to new theories or amendments to existing ones. The piezoelectric effect, the core principle that operates certain sensors and actuators, has led to substantial developments in solid-state physics and material science. Moreover, transduction symbolises a quintessential arrow in time since it dictates a key directionality to energy flow - from higher to lower energetic states. This intrinsic link between time and energy flow is a pivotal concept in understanding entropy and thermodynamics. Additionally, the concept of irreversibility associated with energy dissipation has had profound implications in statistical physics and cosmology, with potential influences on theories like the 'arrow of time' and 'heat death of the universe'. Transduction, in essence, holds a mirror to the crux of many physical phenomena. Every nuance, every shift observed in a transduction process contributes a piece to the grand jigsaw puzzle that physics diligently solves - unravelling the workings of the universe.Analysing Mechanisms of Transduction
Transduction in physics plays a pivotal role as it bridges the chasm between different energy forms, fostering numerous technological innovations. The crux of this interdisciplinary impact lies in understanding the inherent mechanisms at work in transduction. Each transducer, depending on its design and the type of energy transformation involved, operates on a certain mechanism that governs how input energy morphs into output energy. Analysing these mechanisms can unlock a vast panorama of energy transformations, guiding novel solutions and applications.Fundamental Mechanisms in Transduction
The fundamental mechanisms that drive transduction range from simple motion to complex interactions at quantum levels. Yet, at the heart of every mechanism is a straightforward principle of energy transformation — transitioning from one form of energy to another. A brief overview of some mechanisms can reveal the beauty and diversity of transduction:- Mechanical Motion: The simplest form of transduction can be seen in devices such as dynamos or generators, where mechanical motion transforms into electrical energy. The key mechanism here lies in Faraday's law of electromagnetic induction, which states that a change in magnetic environment of a coil of wire induces an electromotive force in the wire.
- Electromagnetic Effect: Devices like transformers rely on electromagnetic interactions to effectuate transduction. A transformer works on the principle of mutual induction, a phenomenon where a change in current through one coil induces a voltage in a neighbouring coil.
- Photoelectric Effect: This effect lies at the core of devices like solar panels. The photoelectric effect refers to the emission of electrons from a metal when light of a certain frequency shines on it. This conversion of light energy into electricity forms the backbone of photovoltaic technology.
- Thermo-Electric Effect: Certain materials show a unique ability to generate an electric potential difference when subjected to a temperature gradient. This principle of thermoelectricity paves the way for devices like thermocouples and Peltier coolers, transforming thermal energy into electrical energy or vice versa.
How Transduction Mechanisms Impact Physics Studies
Comprehensive understanding of transduction mechanisms comes with broad-reaching implications in various fields of physics. The existence, variety, and inherent properties of these mechanisms often open novel avenues of theory, research, and application. Study of transducers and their mechanisms has greatly contributed to electromagnetism. Concepts like inductance, capacitance, resonance, and impedance, which are crucial in the operation of many transducers, have been the linchpin for technological developments. They bridge the divide between pioneering theories and practical applications, fuelling our understanding of electromagnetic phenomena. Analysing transduction mechanisms also adds valuable insights into material science and solid-state physics. The study of materials forms a cornerstone of transducer technology, playing a significant role in determining efficiency, sensitivity, and practicality of energy conversion. For instance, the discovery of piezoelectricity led to a broader understanding of crystalline structures and their extraordinary capabilities in transduction. The impact of understanding transduction mechanisms strongly resonates in the realm of quantum physics as well. Phenomena such as electron excitation in semiconductors or interactions of photons with matter, employed in photoelectric and photovoltaic effects, have spurred comprehensive research at the quantum level. Exploitation of quantum effects in transduction enables devices like quantum dots and quantum well infrared photodetectors (QWIPs), pushing the boundaries of technological innovation. In summary, the study of transduction mechanisms provides valuable insights into various aspects of physics. It forms the bridge between theoretical concepts and practical devices, significantly propelling technological advancements while continually refining our existing theories and our understanding of nature.Transduction: Its Role and Influence in Modern Physics
Transduction, as a fundamental concept, threads itself throughout the fabric of physics. Its simplicity that lies in the transformation of energy from one form to another belies its suggestive power in shaping and directing the trajectory of modern physics. By understanding energy conversion, new vistas in this field emerge offering insights into the exploration of both macroscopic and microscopic worlds.The Impact and Importance of Transduction in Physics
Transduction plays a pivotal role in the understanding and application of physics. Its importance can be perceived through a wide array of physical phenomena and technological advancements it affects directly and indirectly. In physics, energy is the central quantity of interest. Its sacrosanct status is evident in the law of conservation of energy which states that energy cannot be created or destroyed, just transformed. This transformation is what we know as transduction. The multitude of transduction processes that occur in physical systems serves as an affirmation of the unity of physical phenomena.Law of conservation of energy: This fundamental law in physics posits that energy within an isolated system remains constant. It can only be transformed from one form to another. Represented in mathematical terms, it is usually expressed as: \[ \text{Total Energy}_{\text{initial}} = \text{Total Energy}_{\text{final}} \]
Transduction: An Innovation in Modern Physics Study
In the panoramic vista of knowledge, transduction forms a crucial constituent in modern physics. It serves as a dynamic link between theoretical principles and their practical applications in fields such as electronics, telecommunications and energy sector among others. One of the burgeoning areas where transduction is exhibiting profound influence is in the realm of renewable energy technologies. Solar energy harvesting relies on photovoltaic transducers which employ the photoelectric effect to convert solar energy into electric energy. Development and understanding of efficacious transduction mechanisms are proving instrumental in pushing the boundaries of renewable energy technologies. Advancements in telecommunications and computing technology owe largely to the innovation in transduction mechanisms. High-frequency signal transmission, optic-fibre communication, data storage, and processing technologies all harness unique transduction processes for smooth and efficient operation. It is through a firm grasp of transduction principles that technological developments in these sectors have happened apace.Optic-Fibre Communication: This mode of communication employs transmission of light as a signal through a thin strand of glass, known as optical fibre. Here, transduction happens at both the sender's end, where electrical signals are converted into light signals, and the receiver's end, where the inverse happens.
Transduction - Key takeaways
- Transduction is the process of converting one form of energy into another, following scientifically established laws.
- Examples of transduction in physics include microphones (audio to electrical energy), loudspeakers (electrical to audio energy), and solar panels (solar to electrical energy).
- Key steps in a physics transduction process include input energy, energy conversion, output energy, and performance and efficiency.
- Principle of energy conservation applies to transduction, stating that while energy can change forms, its total amount remains constant in a closed system.
- Specific transduction mechanisms include mechanical motion, the electromagnetic effect, the photoelectric effect, and the thermo-electric effect.
Learn faster with the 15 flashcards about Transduction
Sign up for free to gain access to all our flashcards.
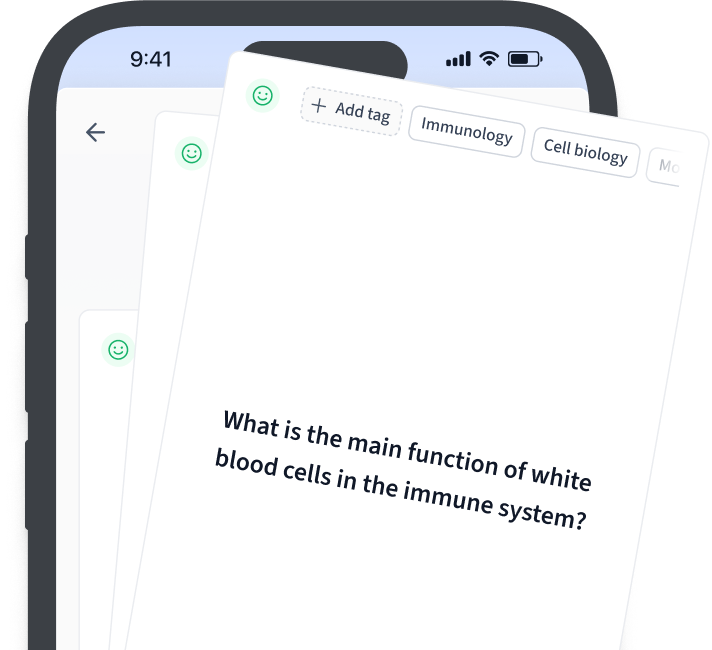
Frequently Asked Questions about Transduction
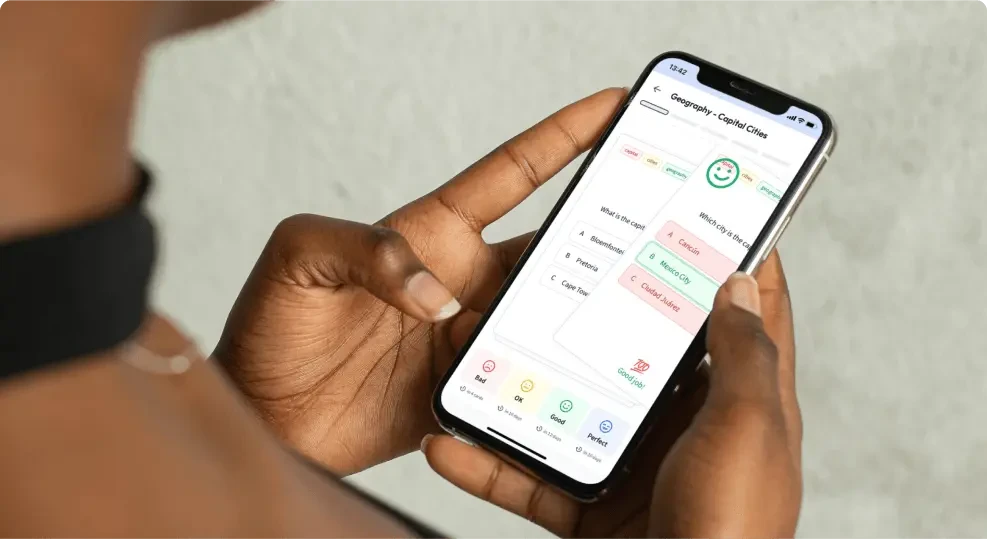
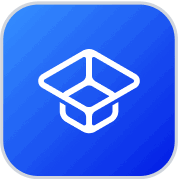
About StudySmarter
StudySmarter is a globally recognized educational technology company, offering a holistic learning platform designed for students of all ages and educational levels. Our platform provides learning support for a wide range of subjects, including STEM, Social Sciences, and Languages and also helps students to successfully master various tests and exams worldwide, such as GCSE, A Level, SAT, ACT, Abitur, and more. We offer an extensive library of learning materials, including interactive flashcards, comprehensive textbook solutions, and detailed explanations. The cutting-edge technology and tools we provide help students create their own learning materials. StudySmarter’s content is not only expert-verified but also regularly updated to ensure accuracy and relevance.
Learn more