Energy in Simple Harmonic Motion
We remember that in order for a system to complete one oscillation cycle in simple harmonic motion, the object is initially released from some displacement from the equilibrium position, passes through the equilibrium position, reaches the maximum displacement at the other end, and passes again through the equilibrium position before returning to its starting point. At maximum displacement from the equilibrium point, the potential energy is at its maximum while its kinetic energy is equal to
Fig 1 - Conservation of energy in SHM Systems. We can see energy is interchanging between potential energy and kinetic energy in every moment of the oscillating motion.
Potential Energy in Simple Harmonic Motion
We consider a spring-mass system to find the potential energy expression in an example of simple harmonic motion. The spring force is a conservative force, so we can define the potential energy for it. As a quick reminder, a conservative force is any force that is independent of an object's path, such that it depends only on the displacement of the object. The spring's potential energy is the energy accumulated in the spring when it is compressed. To find the expression, we can determine the work done by the force solving an integral or, more easily, we can plot the spring force as a function of position and determine the area under the curve.
Fig 2 - Spring force as a function of position to illustrate how to determine the spring's potential energy by calculating the area below the curve.
From the above figure, we see that the area under the curve is a triangle. So, we can easily determine the expression of the spring's potential energy:
where
We know the conservative force that acts on the spring-mass system, so we can solve the integral to find the potential energy stored in the spring.
Kinetic Energy in Simple Harmonic Motion
Now that we know energy is conserved in simple harmonic motion, and we have the expression for the potential energy, we can determine the expression for velocity, as we know the equation for the kinetic energy:
Initially, we are at maximum displacement so,
Now that we know the expression for the velocity of the object undergoing simple harmonic motion, we can determine the equation for the kinetic energy of simple harmonic oscillators:
Total Mechanical Energy in Simple Harmonic Motion
Now that we know the expressions for kinetic and potential energy in simple harmonic motion, we can determine the equation for the total mechanical energy of a simple harmonic oscillator.
Consider a spring with spring constant
Another way to visualize the total mechanical energy is to think in terms of the expressions for the position and velocity of a simple harmonic oscillator given by
If we examine the energy of the system, we see that the potential energy oscillates as a cosine-squared function, while the kinetic energy oscillates as a sine-squared function.
where the trigonometric identity
So, we see the total mechanical energy of the simple harmonic oscillator is the sum of the kinetic energy of the mass and the potential energy stored in the spring. The energy of the system is proportional to the square of the amplitude, such that the amplitude affects the total energy. Also, we see that the total energy in this system is constant over time.
A block of mass
When the block is at amplitude position, the kinetic energy is zero.
To determine the expression for the velocity, we solve for
So at
Mechanical Energy in SHM Systems - Key takeaways
- Energy is constant and conserved in simple harmonic motion.
- At maximum displacement from the equilibrium point, the potential energy is at its maximum while kinetic energy is zero, as the velocity on this moment is zero.
At the equilibrium point, the potential energy is zero and the kinetic energy is a maximum.
The spring force is a conservative force, so we can define a potential energy for it.
A conservative force is any force that is independent of an object's path, such that it depends only on the displacement of the object.
We can plot the spring force as a function of position and determine the area under the curve to get the equation for potential energy. The area of this curve is a triangle.
The spring's potential energy is
.The kinetic energy of the spring-mass system is
.The total mechanical energy of the simple harmonic oscillator is the sum of the kinetic energy of the mass and the potential energy stored in the spring
The energy of the system is proportional to the square of the amplitude, such that the amplitude affects the total energy,
.
Learn faster with the 15 flashcards about Mechanical Energy in Simple Harmonic Motion
Sign up for free to gain access to all our flashcards.
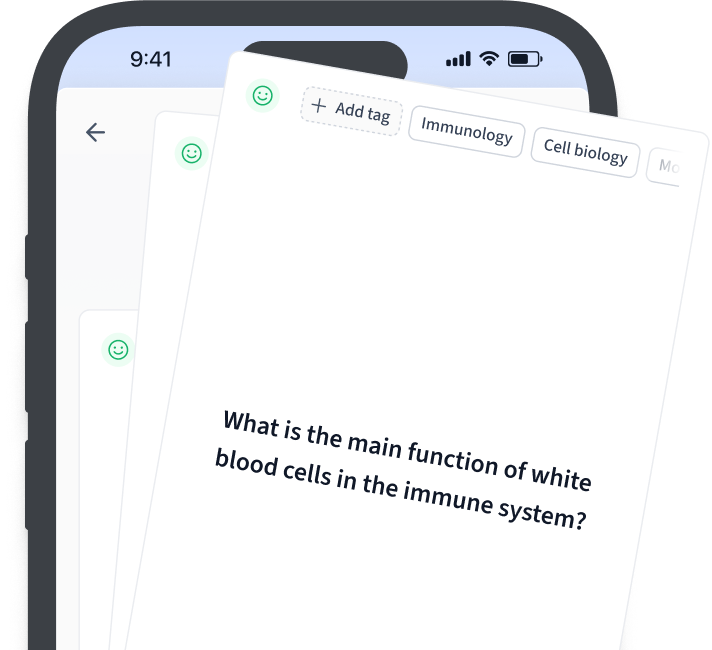
Frequently Asked Questions about Mechanical Energy in Simple Harmonic Motion
How do you find total mechanical energy in simple harmonic motion?
To find the total mechanical energy in simple harmonic motion you must know the value for the spring constant and the amplitude of oscillation, .
Is total mechanical energy constant in simple harmonic motion?
Energy is constant and conserved in simple harmonic motion.
Why is mechanical energy always conserved in an ideal simple harmonic oscillator?
Conservation of energy implies that the total energy of a system undergoing simple harmonic motion is constant. That means that energy in these systems is always interchanging between potential and kinetic energy. When the potential energy is at its lowest, the kinetic energy of the system is at its highest, and viceversa.
What is called mechanical energy of a particle executing simple harmonic motion?
It is the total energy of the system, and the sum of the kinetic energy of the particle and the potential energy caused by the force acting against the particle.
How do you find maximum kinetic energy in simple harmonic motion?
At the equilibrium point the potential energy is zero and the kinetic energy is a maximum, as we can see from its equation .
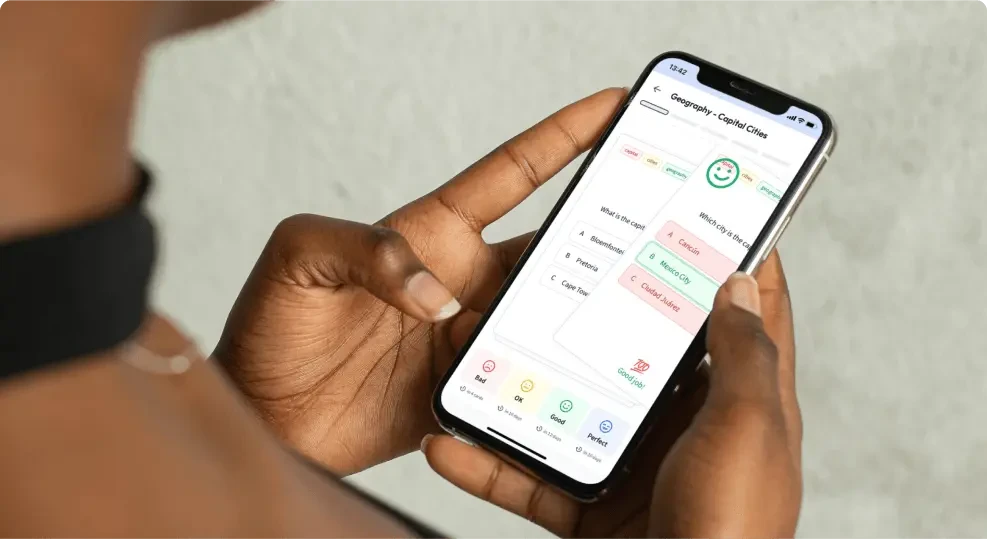
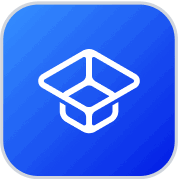
About StudySmarter
StudySmarter is a globally recognized educational technology company, offering a holistic learning platform designed for students of all ages and educational levels. Our platform provides learning support for a wide range of subjects, including STEM, Social Sciences, and Languages and also helps students to successfully master various tests and exams worldwide, such as GCSE, A Level, SAT, ACT, Abitur, and more. We offer an extensive library of learning materials, including interactive flashcards, comprehensive textbook solutions, and detailed explanations. The cutting-edge technology and tools we provide help students create their own learning materials. StudySmarter’s content is not only expert-verified but also regularly updated to ensure accuracy and relevance.
Learn more