Jump to a key chapter
Understanding Isotopes and Radiation
Learning about isotopes and radiation can open up a whole new view on the way you perceive the universe. Isotopes and radiation are two key concepts in the world of Physics, and they are interconnected in many ways. To have a clear understanding, let's explore these concepts individually and learn about their connection.
Definition: What is Isotope Radiation?
Isotope radiation, also known as radioactive decay, refers to the process by which an unstable atomic nucleus loses energy by emitting radiation. This radiation can take the form of alpha particles, beta particles, gamma rays, or other particles.
Radiation can be emitted by isotopes (versions of an element with different numbers of neutrons in their nuclei) when they are radioactive. This happens when the isotopes are unstable and release radiation in an attempt to become more stable. The radiation emitted due to this process is what we call isotope radiation.
For example, Carbon-14, a radioactive isotope of carbon, emits beta particles during its radioactive decay. This is a widely known example of isotope radiation, especially significant in the field of archaeological dating, known as radiocarbon dating.
Isotope Radiation Examples for clearer understanding
To understand isotope radiation better, let's look at some specific examples involving different types of isotopes and the radiation they emit.
Isotope | Type of Radiation Emitted |
Uranium-238 | Alpha particles |
Carbon-14 | Beta particles |
Cobalt-60 | Gamma rays |
In the examples above, each radioactive isotope emits a specific type of radiation. Uranium-238, largely present in Earth's crust, emits alpha particles as a part of its radioactive decay chain. Carbon-14, as mentioned earlier, emits beta particles, and it's crucial in the dating of archaeological samples. Cobalt-60, product of specific nuclear reactions, emits highly energetic gamma rays, making it useful but also dangerous if not handled properly.
Did you know that radiation therapy for treating cancer often relies on isotopes, like Cobalt-60, to deliver targeted doses of radiation to kill cancer cells? This therapeutic use of isotope radiation emphasizes the duality of radiation – it can be extremely harmful, yet it can also be used in medical treatments that save lives.
Understanding isotopes and their associated radiation helps in various scientific fields, from archaeology and medical science to nuclear energy production and much more. It's a vast and fascinating topic, and the knowledge you gain is sure to assist you not just in your physics coursework, but also in comprehending the world at a fundamental level.
Why do Radioactive Isotopes Emit Radiation?
The world of radioactive isotopes is both fascinating and complex. To understand why these isotopes emit radiation, you need to delve into the heart of the atoms themselves, exploring their basic structures and how their components interact with each other. The key lies in the stability of the atomic nucleus.
Unpacking the science behind Radioactive Isotopes
Atoms are composed of protons, neutrons, and electrons. Protons and neutrons reside in the nucleus at the centre of the atom, while electrons orbit this nucleus in shells.
Radioactive isotopes, or radioisotopes, are variants of chemical elements that possess an unstable nucleus. This instability arises from an excess of neutrons or deficit of neutrons in the nucleus, unbalancing the atom.
In a bid to achieve a more stable state, these radioactive isotopes undergo a process called radioactive decay, spontaneously emitting radiation in the form of particles and/or energy. This process transforms one element to another and continues until a stable isotope is formed.
For instance, think about Uranium-238, a well-known radioactive isotope. It undergoes a decay chain that leads to the formation of a stable lead isotope (Pb-206), emitting alpha and beta particles along the way. The equation evolved is \[{}^{238}_{92}U \rightarrow {}^{206}_{82}Pb + 8 {}^{4}_{2}He + 6 {}^{0}_{-1}e \]. Each \( {}^{4}_{2}He \) represents an alpha particle and each \( {}^{0}_{-1}e \) a beta particle.
The types of radiation emitted during this decay process can be alpha particles (consisting of two protons and two neutrons), beta particles (either an electron or a positron), or gamma rays (a form of electromagnetic radiation similar to x-rays).
- Alpha Decay: Occurs when an isotope emits an alpha particle. This results in a new atom with two fewer protons and two fewer neutrons.
- Beta Decay: Involves the transformation of a neutron into a proton while emitting an electron (beta negative decay) or the transformation of a proton into a neutron with the emission of a positron (beta positive decay).
- Gamma Decay: Usually follows alpha or beta decay, where the remaining energy is used to emit a highly energetic photon known as a gamma-ray.
Analysis of Unstable Isotopes
Unstable isotopes are at the heart of our discussion of radioactive decay. These isotopes have an imbalance in their atomic structure, leading to the emission of radiation as the isotope seeks stability.
An unstable isotope is an isotope whose nucleus does not have enough binding energy to hold the nucleus together. Consequently, the nucleus decays and changes to another atom, a process accompanied by emission of radiation.
Such a decay can be represented by the formula \[\text{{parent nucleus}} \rightarrow \text{{daughter nucleus}} + \text{{radiation}}\].
Until it turns into a stable daughter nucleus, unstable isotopes often have a sequence of reactions, producing a chain of elements between itself and the final product. This sequence is known as a decay chain.
Understanding the Role of Stable Isotopes
While some isotopes are unstable and undergo radioactive decay, others are stable and do not generally change over time. These are known as stable isotopes.
A stable isotope is an isotope that has a perfectly balanced nucleus and does not spontaneously undergo any change over time.
Stable isotopes have a wide range of applications. For example, in chemistry they are used for tracing the path of atoms through chemical reactions. In environmental studies, they help in understanding climate change. In health science, stable isotopes of hydrogen and oxygen are used to measure total body water and therefore human energy expenditure.
In carbon dating, Carbon-12 (a stable isotope) and Carbon-14 (a radioactive isotope) are used together. Living organisms continually exchange carbon with the atmosphere through the process of photosynthesis or through food chains. Upon death, this exchange stops, and while the amount of stable Carbon-12 remains constant, the radioactive Carbon-14 decays. By measuring the ratio of Carbon-14 to Carbon-12 remaining in the organism, the time that has passed since death can be calculated.
Different Radiation Types and Their Sources
Understanding radiation requires comprehension of its diverse types and their origins. Broadly, the types of radiation can be categorised into ionising and non-ionising, but for the purposes of isotopes and radioactive decay, focus is on ionising radiation, particularly alpha, beta and gamma radiations.
How Different Isotopes Emit Different Types of Radiation
Every isotope is unique with its specific number of protons and neutrons, leading to inherent stability or instability. The type of radiation emitted by a radioactive isotope depends predominantly on this nuclear composition and the nature of instability. Let's take a closer look at how different isotopes emit different types of radiation.
Unstable isotopes erratically release energy to attain stability. This energy is released as radiation, consisting of charged particles or high-energy photons. The type of radiation is dependent on the nature of decay and the particular imbalance in the atomic nucleus.
Radioactive decay is the process wherein an unstable atomic nucleus loses its excess energy by emitting radiation in the form of particles or electromagnetic waves.
- Alpha Decay: Alpha decay happens when an isotope emits an alpha particle which has two protons and two neutrons. Hence, the daughter atom formed has two fewer protons and two fewer neutrons than the parent atom.
- Beta Decay: Beta decay occurs when a neutron inside the nucleus transforms into a proton, releasing an electron in the process, or conversely, when a proton turns into a neutron, emitting a positron.
- Gamma Decay: In gamma decay, the nucleus transitions from an excited state to a lower energy state and emits a gamma ray. Unlike alpha and beta decays, no change in atoms occurs, only the energy state.
Consider the isotope Uranium-238. This isotope is prone to alpha decay, transforming into Thorium-234 and emitting an alpha particle in the process. The decay can be represented as: \({}^{238}_{92}U \rightarrow {}^{234}_{90}Th + {}^{4}_{2}He\). Here, \( {}^{238}_{92}U \) is Uranium-238 (parent nucleus), \( {}^{234}_{90}Th \) is Thorium-234 (daughter nucleus), and \( {}^{4}_{2}He \) represents an alpha particle.
It's fascinating to note that the product of one radioactive decay can itself be radioactive and undergo further decay, setting off a chain of emissions until a stable isotope is finally formed. This sequence is known as a radioactive decay chain.
Radioactive Isotopes and Gamma Radiation
Gamma radiation is dissimilar to alpha and beta radiation in that it's an electromagnetic wave, akin to X-rays, but with much higher energy. This radiation is emitted when the nuclear particles arrange themselves into a more stable configuration after a nuclear reaction or radioactive decay, resulting in excess energy being emitted.
Gamma radiation or gamma rays are extremely high-energy photons emitted by an atomic nucleus during a nuclear reaction or radioactive decay.
A prominent example of a gamma emitter is Technetium-99m, a metastable nuclear isomer used in various types of medical imaging. Its decay can be represented as \[ {}^{99m}_{43}Tc \rightarrow {}^{99}_{43}Tc + \gamma \], where \(\gamma\) represents the emitted gamma radiation.
Beta Radiation Examples in Isotopes
Beta decay is a unique phenomenon involving the transformation of a neutron into a proton, or vice versa, inside the nucleus. This conversion results in the emission of an electron (beta negative decay) or a positron (beta positive decay), and a corresponding neutrino or antineutrino.
Beta radiation refers to the high-speed electrons or positrons emitted by certain types of radioactive nuclei such as potassium-40 and carbon-14.
A classic example of an isotope emitting beta radiation is carbon-14. It undergoes beta minus decay to produce nitrogen-14, emitting an electron and an electron antineutrino in the process, expressed as \( {}^{14}_{6}C \rightarrow {}^{14}_{7}N + {}^{0}_{-1}\beta + \overline{\nu} \), where \( {}^{0}_{-1}\beta \) is an electron and \( \overline{\nu} \) is an electron antineutrino.
Alpha Radiation: How it Links to Isotopes
Alpha radiation is fundamentally the emission of alpha particles, each consisting of two protons and two neutrons, essentially a helium-4 nucleus. This type of radiation is commonly associated with heavy isotopes, such as those of uranium and thorium.
An alpha particle is a type of ionising radiation consisting of two protons and two neutrons. It is essentially a helium-4 nucleus and is emitted in the alpha decay process.
For instance, uranium-238, a naturally occurring isotope found in the Earth's crust, undergoes alpha decay to form thorium-234, represented as \( {}^{238}_{92}U \rightarrow {}^{234}_{90}Th + {}^{4}_{2}He \). This conversion subsequently kicks off a series of decays known as the uranium decay series.
Following the transition mentioned above, Thorium-234 itself is unstable and undergoes beta decay forming Protactinium-234, which also decays by beta emission to form Uranium-234, and so on. This chain of decays continues until stable Lead-206 is formed. During these series of decays alpha, beta and gamma radiations are all emitted signifying that a radioactive isotope can emit more than one kind of radiation.
Real Life Applications of Isotopes Radiation
Isotopic radiation, intriguing as it may be in its scientific underpinnings, is not just confined to textbooks and laboratories. Various real-world applications leverage isotopic radiation. From healthcare and energy production to scientific research and archaeology, its role is deeply woven into myriad facets of modern life.
Practical Examples of Isotopes Radiation in use
Isotopes and their radioactive decay have found extensive applications across a multitude of fields. They provide crucial knowledge and practical utilities in medicine, genetic engineering, power generation, archaeological dating, smoke detection, and several other areas.
- Medical Imaging: Radioactive isotopes are used in various diagnostic techniques, including PET scans and bone scans. For instance, Technetium-99m is widely used as a tracer in such imaging procedures.
- Power Production: Uranium-235 and Plutonium-239, due to their capacity to undergo controlled chain reactions, are regularly used as fuel in nuclear fission reactors for electricity generation.
- Radiocarbon Dating: The decay of Carbon-14, a radioactive isotope of carbon, is useful in determining the age of archaeological and geological samples.
- Genetic Studies: Isotopes are commonly used as tracers in genetic and biochemical investigations to reveal metabolic and chemical processes in organisms.
All these uses arise from the fundamental fact that isotopes of the same element behave in the same way chemically, but their radioactive properties enable us to track or trace them with ease.
The Use of Isotopes in the Medical Field
The medical field perhaps exhibits the most significant application of isotopic radiation. Radioactive isotopes have far-reaching utilities in both diagnostic and therapeutic procedures.
Radiopharmaceuticals are drugs that contain radioactive isotopes. These drugs are used in the diagnosis and treatment of many diseases. The isotopes emit radiation that can be detected by specific devices, creating images of the inside of a patient's body, or delivering targeted radiation to treat diseases like cancer.
Technetium-99m, as aforementioned, is a shining example of a radionuclide commonly used for producing gamma radiation in diagnostic imaging. Research has also ushered in the use of beta emitters like Yttrium-90 and Lutetium-177 for targeted cancer therapy, often referred to as radionuclide therapy.
Further, isotopes with short half-lives are preferred for diagnostic procedures to limit the patient's exposure to radiation. On the contrary, isotopes with longer half-lives are selected for therapeutic interventions to ensure prolonged emission of radiation for destroying harmful cells.
For example, iodine-131 is a radioactive isotope utilised in both the diagnosis and treatment of thyroid conditions. When ingested, it preferentially accumulates in the thyroid gland. The emitted gamma radiation is then detected to reveal the size, shape, and activity of the thyroid, making it effective for diagnosis. Its beta radiation, on the other hand, can destroy overactive thyroid tissue or thyroid cancer cells, illustrating its therapeutic use.
How Isotopes Radiation is Utilised in Scientific Research
Scientific research widely harnesses isotopic radiation, spanning across disciplines like physics, chemistry, geology, biology, and environmental science.
A tracer is a substance that can be detected while moving through a system. Radioisotopes, owing to their detectable radiation, are excellent tracers. They allow scientists to trace the path or transformation of an element in a system, providing invaluable insights into complex physical, chemical, or biological processes.
The decay of isotopes allows scientists to read the past. For instance, the method of radiocarbon dating, wherein the radioactive decay of Carbon-14 enables the determination of the age of ancient organic materials, is a classic showcase of isotopic radiation in action.
Furthermore, stable isotopes like Oxygen-18 and Deuterium are crucial in studying earth's climate history, while isotopic tracers help discern the intricacies of processes like photosynthesis, nutrient cycling, and ocean circulation.
Let's consider the instrumental role carbon isotopes play in carbon dating. When a plant or animal dies, the radiocarbon or Carbon-14 it contains starts to decay at a known rate, while the amount of stable Carbon-12 remains constant. By measuring the current ratio of Carbon-14 to Carbon-12 in fossilized remains and knowing the half-life of Carbon-14 (about 5730 years), scientists can deduce the time that has elapsed since the death of the organism, thereby estimating the age of the sample.
Did you know that banana plants take up the isotope Potassium-40, a naturally radioactive isotope, from the soil? So, human exposure to this isotope radiation occurs every day, even from eating a banana! Albeit, the number of decays is minuscule and poses no threat to health. This fascinating example illustrates that we live in a naturally radioactive world.
Diving Deeper into Isotopes Radiation
To delve deeper into isotopes radiation, it's essential to understand the underlying science that dictates their behaviour. This particularly involves the stability of isotopes and their radioactive tendencies. Stable and unstable isotopes form the bedrock of our discourse on atomic structure and radiation phenomena. Hence, a comprehensive understanding of these will allow you to better comprehend the broader topics of nuclear physics.
The Science Behind Stable and Unstable Isotopes
What makes an isotope stable or unstable lies at the heart of the nucleus - the protons and neutrons. Their numbers, alignment, and the forces amongst them dictate the stability or lack thereof.
An isotope is a form of an element characterised by a specific number of neutrons in its nucleus. Depending on the balance of nuclear forces within, an isotope may be stable or unstable. Stable isotopes have a balanced nucleus and do not undergo radioactive decay. Unstable isotopes, also known as radioisotopes, contain an imbalanced nucleus and hence, undergo radioactive decay, emitting radiation.
In the realm of isotopes, the strong nuclear force and the electromagnetic force play a crucial role. The strong nuclear force binds the protons and neutrons together, while the electromagnetic force causes repulsion between protons due to their similar positive charges. A balance between these forces leads to a stable nucleus. Conversely, an imbalance leads to instability, driving the isotope to seek balance by emitting radiation, fact known as radioactive decay.
Consider Carbon-12, a stable isotope of carbon. It has six protons and six neutrons in its nucleus. The balanced forces within the nucleus confer stability. On the other hand, Carbon-14, an unstable isotope of carbon, has six protons and eight neutrons. This neutron excess creates an internal imbalance, causing the isotope to undergo beta decay. The decay process results in a stable Nitrogen-14 isotope alongside the release of a beta particle and an electron antineutrino.
What makes an Isotope Stable or Unstable?
The question of what determines an isotope's stability is a complex one, but fundamentally it rests on the nature and balance of the forces within an atomic nucleus. The primary forces at play within the nucleus are the repulsive electromagnetic force among protons and the attractive strong nuclear force between protons and neutrons.
An isotope's stability is primarily determined by the balance of forces in its nucleus. A nucleus where the repulsive electromagnetic force (due to protons) is counterbalanced by the attractive strong nuclear force (between protons and neutrons) is considered stable. A nucleus where these forces are imbalanced is unstable and seeks stability through radioactive decay, resulting in isotopes radiation.
The proton to neutron ratio plays a significant role in this stability. For lighter elements (atomic number up to 20), stability is generally observed when the number of protons equals the number of neutrons. For heavier elements, a greater number of neutrons is required to achieve nuclear stability due to the increased repulsion between the larger number of protons.
How Radiation Relates to Isotope Stability
Radiation is a direct outcome of an isotope's quest for stability. When a radioactive isotope possesses a surplus or deficit of neutrons, making its nucleus unstable, it undergoes a spontaneous process called radioactive decay, seeking a more stable state.
Radioactive decay refers to the transformation of an unstable nucleus into a more stable one through the release of energy in the form of radiation. This can result in the emission of alpha particles, beta particles, or gamma rays, subject to the nature of decay and the specific isotope involved.
In the process of radioactive decay, the parent nucleus transforms into a daughter nucleus, emitting radiation in the process. The equation for such a decay is generally represented as \[\text{{parent nucleus}} \rightarrow \text{{daughter nucleus}} + \text{{radiation}}\]. The decay continues until a stable isotope is formed.
Plutonium-239 provides an interesting example. It undergoes alpha decay, emitting an alpha particle (helium nucleus) and transforming into Uranium-235. The generic representation would be \( {}^{239}_{94}Pu \rightarrow {}^{235}_{92}U + {}^{4}_{2}He \). Over many such decays, this process eventually leads to the formation of a stable isotope of lead (Pb).
Interestingly, some isotopes exist in a 'metastable' state. They are technically excited states of a stable or unstable nucleus and can stay in this state for an extended period before transitioning to a lower energy state, emitting gamma radiation in the process. Technetium-99m, widely used in nuclear medicine, stands as a classic example of such isotopes.
Isotopes Radiation - Key takeaways
- Isotopes Radiation: Isotopes, either stable or unstable, interact with their environment through radiation. Unstable isotopes reach stability through alpha, beta or gamma radiation, whereas stable isotopes remain unchanging over time.
- Alpha Decay: A type of radioactive decay in which an alpha particle (two protons and two neutrons) is emitted, resulting in a new atom with two fewer protons and two fewer neutrons.
- Beta Decay: A type of radioactive decay involving the transformation of a neutron into a proton, or vice versa, inside the nucleus. During this decay process either an electron or a positron is emitted.
- Gamma Decay: Occurs following alpha or beta decay, where the remaining energy is used to emit a highly energetic photon, the gamma-ray, without any change in the atoms.
- Unstable and Stable Isotopes: Unstable isotopes have an imbalance in their atomic structure, which causes them to emit radiation as they seek stability. Stable isotopes however, have a perfectly balanced nucleus and do not spontaneously undergo any change over time.
Learn faster with the 19 flashcards about Isotopes Radiation
Sign up for free to gain access to all our flashcards.
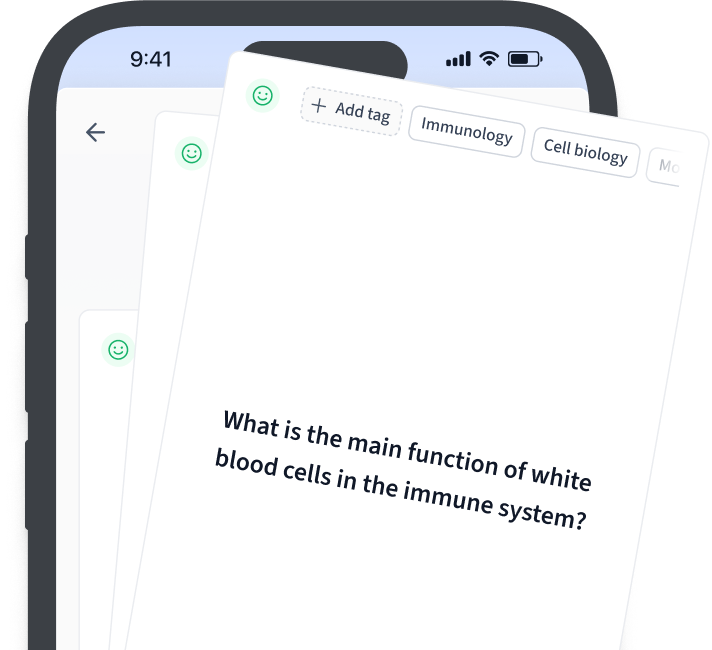
Frequently Asked Questions about Isotopes Radiation
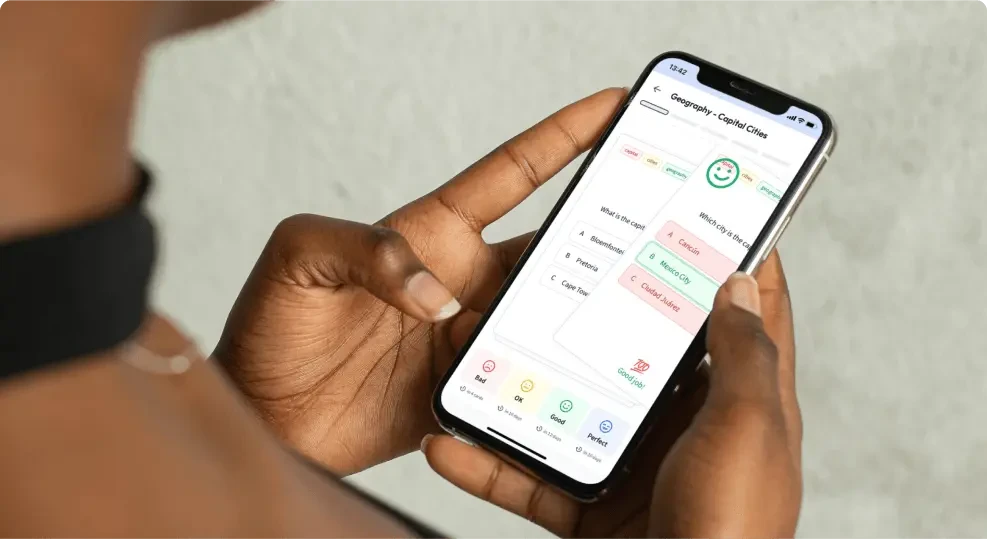
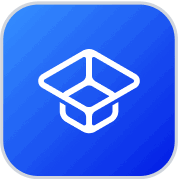
About StudySmarter
StudySmarter is a globally recognized educational technology company, offering a holistic learning platform designed for students of all ages and educational levels. Our platform provides learning support for a wide range of subjects, including STEM, Social Sciences, and Languages and also helps students to successfully master various tests and exams worldwide, such as GCSE, A Level, SAT, ACT, Abitur, and more. We offer an extensive library of learning materials, including interactive flashcards, comprehensive textbook solutions, and detailed explanations. The cutting-edge technology and tools we provide help students create their own learning materials. StudySmarter’s content is not only expert-verified but also regularly updated to ensure accuracy and relevance.
Learn more