Jump to a key chapter
Understanding the Random Coil in Physics
The fascinating world of physics extends beyond planed trajectories and predictable systems, encompassing structures that emerge randomly without fixed form or discernible pattern. An instance of this concept is represented in the 'random coil', a term you may encounter in studies related to polymer science, materials physics, and biophysics.
Random Coil Definition and Context in Physics
A random coil is a conformation of a long-chain molecule, such as a polymer or protein, where the positions of the constituent atoms follow no discernible order. This structure appears derives its name from its unpredictable, non-repetitive configuration.
The attributes of a random coil in physics are predominantly influenced by the thermodynamic conditions and the internal properties of the molecule in question. Changes in temperature, pressure, or solvent environment can trigger a transition from a random coil state to a more structured conformation such as an alpha helix in proteins, or vice versa.
For instance, think of a freely jointed polymer chain in a solvent. Without any external forces or intermolecular attractions, the polymer chain adopts a random coil conformation where every segment orientation is equally probable and independent of others. This randomness can be modeled by statistical methods, giving us insight into many polymer properties.
Comprehensive Explanation on Random Coil Definition
Doing justice to the random coil concept requires a deep dive into the unpredictability of these structures.
The lack of order in a random coil stems from thermal fluctuations that incessantly disturb the molecule's configuration. This constant jiggle prevents these long-chain molecules from settling into a fixed, ordered structure, giving rise to the 'random' in random coil.
Although the configuration is random, it must be emphasised that this doesn't necessarily imply a lack of structure. Parts of the molecule might habitually form local structures like helices, but the molecule as a whole doesn't keep a fixed form. Ultimately, a random coil represents a balance between entropy favoring disorder and the molecule's internal rules and interactions.
Key Aspects of Random Coil Conformation
- The random coil is a dynamic shapeless structure, continually changing due to thermal vibrations.
- It emerges as the default state when there are no forces compelling the molecule towards a more ordered form.
- The molecule's size, flexibility, and intersegment attractions affect the nature of the random coil state.
Imagine a 'bead on a string' model, where the beads represent segments of the molecule, and the strings represent flexible bonds between them. Each bead can rotate without affecting the others, which illustrates how a molecule can adopt a plethora of conformations collectively called a random coil.
Pertinence of Random Coil Conformation in Solid State Physics
In the realm of solid-state physics, random coil conformations provide indispensable insight into understanding the mechanical properties of materials, such as elasticity and toughness, that are made of interconnected chains. The mathematical representation of a random coil is described as follows:
\[ R=\sqrt{nl^{2}} \]Where \( R \) is the end-to-end distance, \( n \) is the number of segments, and \( l \) is the length of each segment.
Studying physical systems that contain random coils provides a direct way to probe the behaviour of molecules that are at the mercy of thermal fluctuations and their own internal dynamics.
Common Instances of Random Coil Conformation
Random coil conformations are frequently observed in natural and synthetic polymers in solution, proteins under denaturing conditions, and emerging nanomaterials such as graphene oxide. The table below gives a few examples:
Natural Polymers | DNA, Proteins |
Synthetic Polymers | Polystyrene, Polyethylene |
Nanomaterials | Graphene Oxide |
The adoption of random coil conformation essentially acts as a compromise between behaving like rigid crystals or fluid-like gases. This balance offers robustness along with adaptability, crucial for biological systems and versatile materials.
The Role of Random Coil in Protein Structure
In the intricate world of protein structure, one may often associate proteins with classic structures such as alpha helices and beta sheets. However, one must not overlook the importance and influence of the random coil structure. As with other biomacromolecules, proteins often contain regions that do not adopt a defined secondary structure due to various factors, and these are referred to as random coil segments.
Examination of Random Coil in Proteins
The study of random coil segments within proteins is a dynamic and vital component of protein research, occupying a significant place in structural biology. Recall the definition of a random coil as a segment of a long-chain molecule that lacks discernible order and appears random. This randomness is an essential feature of proteins.
Importance of Random Coils in Proteins:
- Random coil segments offer proteins much-needed flexibility, allowing them to interact with other molecules, which is crucial for their function.
- While structured regions of proteins are vital for stability, random coils contribute to the dynamism in protein structure, enabling functionality as enzymes, binders, and more.
- Random coil regions are significant in terms of protein folding dynamics, often serving as transient intermediates. They also act as potential signal carriers for various cell activities.
For quantifying the presence of random coil, one can use experimental techniques, such as X-ray crystallography, NMR spectroscopy, and computational methods like molecular dynamics simulations.
Impact of Random Coil on Protein Structure
The impact of random coils on protein structure is multi-faceted. These regions play a pivotal role in dictating the overall shape and function of the protein. Random coil regions influence the protein's flexibility, define its geometry, and contribute to its stability. Additionally, they act as linkers, allowing different secondary structural elements within a protein to interact.
Interestingly, the same building blocks compose both structured regions and the random coil regions in a protein – amino acids. However, the arrangement and interaction of these amino acids contribute to forming either an ordered structure like an alpha helix or a more flexible random coil region.
The number of random coil segments, their length, and their position within the protein all significantly influence the protein's final structure. Short random coils can provide hinge-like rotations between two structured domains, like links in a chain. Long random coils, on the other hand, can provide necessary spacers between different protein regions, dictating how they can interact.
Alpha Helix, Beta Sheet and Random Coil Interactions
While alpha helices and beta sheets are structured and well-defined, random coil regions complement these ordered structures, providing nuances to protein structures. Their interactions contribute to the tertiary structure (three-dimensional shape) of a protein, thus influencing its functional abilities.
Alpha helices, beta sheets, and random coil regions interplay within a protein structure in a balanced and harmonious way, generating the intricate folding patterns typical of proteins. The transitions from one of these structures to another contribute to shape the overall protein architecture, impacting its flexibility, stability, and function.
In summary, the interplay between these different structures represents a symbiotic relationship, where each structure plays a crucial role.
How Random Coil Interacts With Alpha Helix and Beta Sheets
Random coil regions often act as connectors or linkers between ordered secondary structures like alpha helices and beta sheets. These linkers provide the necessary flexibility and orientation to bring different parts of the protein together in a functional whole.
Furthermore, these coils can pave the way for thermodynamically favourable interactions between different secondary elements, thus contributing to the overall stability of the protein structure.
A change in the environment could trigger transitions between random coils, alpha helices, and beta sheets. A common example is the protein denaturation due to heat or pH change, where the ordered structures unravel into random coils.
Understanding the interaction between these structural elements, including the seemingly nebulous, yet vitally important random coils, is fundamental to understanding protein functionality and how inconsistencies can lead to diseases at the molecular level.
Interpreting Random Coil Chemical Shifts
In the wide-ranging area of solid-state physics and related laboratory applications, nuclear magnetic resonance (NMR) plays an essential role in understanding the structure and dynamics of molecules. A key component of this field is the concept of chemical shifts, which take on a particular significance when dealing with random coil conformations. To navigate through this concept and its profound relevance in physics, it's important to understand what chemical shifts are and their implication in the context of random coils.
Introduction to Random Coil Chemical Shifts
In the broad realm of NMR spectroscopy, chemical shifts are changes in the resonance frequency of a nucleus relative to a standard reference. The random coil chemical shift (RCCS) refers to this phenomenon in the context of random coil conformations of macromolecules like proteins or polymers.
Chemical Shift: Chemical shift is the variation in the observed resonance frequency of an atomic nucleus in an NMR spectrum. It arises due to the influence of the local electronic environment, which modifies the magnetic field experienced by the nucleus, leading to a shift in its resonance frequency. The chemical shift is represented in parts per million (ppm).
Random Coil Chemical Shift (RCCS): RCCS represents the chemical shift expected for a specific nucleus in a long-chain molecule when it is in a random coil conformation. It provides a baseline against which deviations due to formation of secondary structures can be compared.
The Significance of Random Coil Chemical Shifts in Physics
Random coil chemical shifts offer a wealth of information about the structure and dynamics of molecules. This is particularly critical for understanding the behaviour of unstructured or disordered proteins, which cannot be analysed effectively using conventional methods like X-ray crystallography or cryo-electron microscopy.
Apart from providing structural information, RCCS can also offer insights into the conformations and dynamics of molecules. For example, the analysis of temporal fluctuations in RCCS can allow scientists to observe the kinetic behaviour of polymers or proteins.
RCCS is an invaluable tool for gauging conformations and monitoring structural changes in macromolecules upon subtle changes in environment, including alterations in temperature, pressure and solvent characteristics. Furthermore, these shifts enable researchers to investigate and decipher the enthalpic and entropic components driving conformational changes.
Therefore, RCCS serves as an indispensable probe in biomolecular physics, materials science, and nanotechnology.
Applying Random Coil Chemical Shifts in Physics
Random coil chemical shifts are applied extensively in the field of NMR spectroscopy to elucidate the conformations of macromolecules including proteins and synthetic polymers. In these applications, RCCS serve to pin-point the regions of macromolecules that don't participate in the formation of ordered structures and remain as flexible and disordered segments.
By comparing changes in chemical shifts relative to the RCCS, scientists are able to deduce the secondary structural elements that a specific segment of the macromolecule might form under different conditions. This information can be crucial for understanding the function of the molecule and how it can be manipulated.
In addition to the above mentioned areas, RCCS find extensive applications in probing the behaviour of unstructured proteins. By determining the regions that behave as random coils, novel therapeutic strategies can be designed to target these proteins and modulate their function.
Practical Examples of Random Coil Chemical Shifts
Random coil chemical shifts are fundamental for enhancing our understanding of a variety of molecular systems. For instance, they are widely used in the field of protein science. Intrinsically disordered proteins (IDPs), which lack a fixed 3D structure and exist as dynamic ensembles of random coil conformations, are of special interest. By analysing the RCCS of these proteins, researchers can obtain insights into their structural attributes, the environmental influences on their structure, and their dynamic behaviour.
For instance, the RCCS values for IDPs are often compared with the theoretical random coil values for the same sequence. Any deviations from the theoretical values can provide insights into the potential structural propensities of the protein at specific segments. In turn, this information can guide scientists in understanding the potential roles of these proteins in biological systems and how they contribute to diseases when their function goes awry.
Another prime example includes assessing the conformations of synthetic polymers in solvents. RCCS can highlight the flexible segments of the macromolecule that could be potential targets for modifying its physical properties. Essentially, random coil chemical shifts continue to play a key role in our quest to decode the intriguing world of complex molecular structures.
Recognising Random Coil Secondary Architectures
Random coil secondary architecture refers to the structure of macromolecules, such as polymers and proteins, when they are not involved in the formation of ordered structures like alpha helices or beta-pleated sheets. Instead, they present an unpredictable, disordered arrangement, hence the term 'random coil'.
An Overview of Random Coil Secondary Structure
Macromolecules like proteins and polymers interact uniquely with their environment to form a myriad of structures. When these molecules are not part of well-organised, ordered structures, they adopt what is known as the random coil secondary structure.
The random coil secondary structure is notable for its uneven, stochastic arrangement. This conformation is not governed by specific rules, as is the case with ordered structures like alpha-helices or beta-sheets in proteins. They are, instead, structured in a way best described as a 'statistical conformation'. The random coil has no fixed shape but represents a dynamic ensemble of conformations rapidly interchanging over time.
Statistical Conformation: In this context, statistical conformation relates to the fact the random coil adopts an ensemble of rapidly interchanging shapes, influenced by thermal motion and the specific chemical environment, rather than a fixed, defined structure.
The study of these random coil structures provides insights into the behaviour of complex biological systems such as proteins and DNA. By applying the principles of nuclear magnetic resonance spectroscopy, physicists can investigate and analyse these random coil secondary structures in a remarkably quantitative manner, deciphering vital biological processes and phenomena.
Identifying Random Coil Secondary Structure in Physics
In order to reliably identify the random coil secondary structure, physicists resort to powerful analytical tools like NMR spectroscopy. One hallmark of random coil structure, observed in NMR spectra, is its chemical shifts. Chemical shifts are sensitive to the molecular environment and can provide essential clues about the macromolecule's structure.
When a molecule is in a random coil conformation, its chemical shifts are expected to fall into a specific range, known as the Random Coil Chemical Shift (RCCS).
Random Coil Chemical Shift (RCCS): RCCS represents the chemical shift expected for a nucleus in a long-chain molecule when it is in a random coil conformation. It aids in identifying regions of the molecule that are not forming ordered structures and remain flexible.
Any deviations from the RCCS can hint at the formation of ordered secondary structures. Therefore, by comparing the observed chemical shifts with the RCCS values, one can discern the portions of a given macromolecule that are in the random coil conformation.
Unique Features of Random Coil Secondary Structure
Random coil secondary structures are defined by their unique set of characteristics, rooted in their lack of organised structure. Features such as flexibility, variable shape, dynamic behavior, and sensitivity to environmental factors make them distinct from ordered secondary structures.
Flexibility: Random coil structures are remarkably flexible, and this flexibility is key to their function in numerous biological processes. For instance, in the context of proteins, these flexible regions can act as hinges, permitting protein domains to move relative to each other.
Variable Shape: Existing in a state of equilibrium between multiple conformations, macromolecules in a random coil state do not have a fixed shape, but constantly reshuffle their form in response to thermal fluctuations and intermolecular interactions.
Dynamic Behavior: Being devoid of a rigid structure, these macromolecules are characterised by a dynamically changing behavior, with conformations constantly interchanging.
Sensitivity to Environmental Factors: Owing to their lack of defined structure, random coils are extremely sensitive to environmental changes like varying temperature, pH, or solvent conditions, which significantly influence their conformational ensemble.
How to Differentiate Random Coil Secondary Structure
Distinguishing random coil secondary structures from ordered ones, such as alpha-helices or beta-sheets, is a task primarily achieved through techniques like NMR spectroscopy. The key differentiating factor lies in the chemical shifts that emerge from these molecules when exposed to an external magnetic field.
Random coil structures are expected to present chemical shifts in a range labelled as RCCS. If the observed chemical shifts deviate significantly from these values, it suggests that the molecule might be forming an ordered structure like an alpha-helix or a beta-sheet, rather than existing as a random coil.
Moreover, random coils are more flexible and dynamic compared to ordered structures. Therefore, if the region of a macromolecule demonstrates high mobility or flexibility, evaluated through techniques like hydrogen-deuterium exchange or spin relaxation experiments, it might suggest that the segment is in a random coil state.
Indeed, random coil secondary structures exhibit unique thermodynamic, kinetic, and structural properties that merit deep investigation. This structural form is dynamic, flexible, and critically important to many biological processes. It presents both significant challenges and opportunities in the study of macromolecular structures - enabling us to decode the intricate architectural marvels of the molecular world.
Practical Illustrations of the Random Coil in Physics
The principles governing Random Coil physics aren't merely confined to the academic sphere - they transcend the classroom, finding remarkable applications in everyday scenarios. To grasp the full extent of Random Coil physics, it's essential to study its practical illustrations and comprehend its impact in commonplace applications.
Random Coil Physics Example in Everyday Applications
The physics of Random Coils manifests significantly in numerous everyday applications. Let's illustrate this with a representative, easy-to-understand example - making spaghetti.
If you have ever cooked spaghetti, you would have noticed that when you submerge it in boiling water, it turns from being rigid and straight to being flexible and wiggly. The spaghetti noodles, consisting of long-chain macromolecules that start out orderly and determined, quickly take on a more unpredictable, flexible configuration.
Before you cook spaghetti (uncooked state), it exhibits behaviours of an ordered polypeptide chain. However, upon being submerged in boiling water (cooked state), it shifts from this organised form into an ensemble of conformations rapidly interchanging over time, akin to a random coil. The change in spaghetti's structural characteristics, from being stiff and brittle to flexible and arbitrary, mirrors the transformation of an ordered polypeptide chain to a Random Coil.
But how does understanding this transformation in the context of Random Coil physics benefit you in a practical sense?
Benefits of Understanding Random Coil Physics Examples
Perceiving the Random Coil physics example discussed above can enhance your understanding of the various transformations that substances undergo. This comprehension could, in fact, be extended to escalating the quality of substances you interact with daily, especially food items. By controlling the cooking temperature, adjusting the pH, or altering other environmental factors, you could possibly modify the texture (and hence the taste) of your food. The possibilities are indeed intriguing!
For instance, understanding the structural transformation of spaghetti could motivate you to experiment with different ways to cook it to achieve your favoured level of flexibility, firmness, or texture. Being aware that spaghetti's structure changes under different conditions gives you the ability to manipulate these conditions to achieve your desired outcome. Through this process, you may discover a novel approach to cooking, resulting in a different taste or texture improving the overall culinary experience.
For instance, having a deep understanding that lowering the temperature causes the spaghetti to be firmer because it slows down the interchanging conformations of its macromolecules, could enable you to adjust your cooking process accordingly to achieve the desired spaghetti texture.
This is an illustration of how understanding random coil physics could have practical applications in the realm of culinary arts - a realm that almost everyone engages with daily.
Comparing Real-life Random Coil Physics Example
Back to the world of physics, a more technical example can be found in the way pharmaceuticals use Random Coil principles. Tablets and capsules often contain polymers in their formulations. By manipulating their structure and behaviour - from an ordered structure to a Random Coil state - scientists can control the drug release rate, ultimately impacting their therapeutic potential.
Let's consider a comparison between the spaghetti and the pharmaceutical example. They both involve long-chain macromolecules - proteins in the case of spaghetti and polymers for pharmaceutical usage. The polymers and proteins in both examples can transition between ordered and random coil states depending on the surrounding environmental conditions. While you can alter spaghetti's texture by changing its molecular configurations, pharmaceutical companies can modify the drug release rate by controlling the polymer structure in their formulations.
The Impact of Random Coil Physics in Everyday Life
By understanding random coil physics, we gain the ability to manipulate the properties of everyday materials around us, ranging from culinary applications, as observed in the cooking of spaghetti, to more scientifically complex applications, such as controlling drug release rates in pharmaceuticals. This highlights the importance and impact of Random Coil physics in our everyday lives.
The principles of Random Coil physics allow pharmaceutical companies to regulate a tablet's disintegration and dissolution in the user's body, controlling the release rate of the medicine. As a patient, understanding this principle may help you comprehend why some drugs need to be taken on an empty stomach and others just post-meals - it all boils down to how the medicines' macromolecules interact with the environments in your body!
In essence, understanding and applying random coil physics isn't merely an academic exercise but lends us a better grasp of our daily environment. From the food we consume, the pharmaceuticals we take, to the natural phenomena around us, Random Coil physics and its principles pervade myriad aspects of life, subtly yet significantly enhancing our understanding of our surrounding world.
Random Coil - Key takeaways
- Random Coil: An unpredictable and unordered region within a protein's structure that plays a significant role in determining the protein's overall shape, flexibility, and stability.
- Random coils often serve as linkers between structured segments of proteins, such as alpha helices and beta sheets, facilitating their interaction and influencing the protein's tertiary structure.
- Random Coil Chemical Shift (RCCS): In the field of nuclear magnetic resonance spectroscopy, RCCS refers to the changes in the resonance frequency of a nucleus in a macromolecule when in a random coil conformation.
- The analysis of RCCS can provide insight into the structure, conformation, and dynamics of molecules. This is particularly useful for studying unstructured or disordered proteins.
- Random Coil Secondary Structure: When macromolecules like proteins are not part of well-organised structures, they adopt the random coil secondary structure. This structure is known for its dynamic, uneven, and stochastic arrangement.
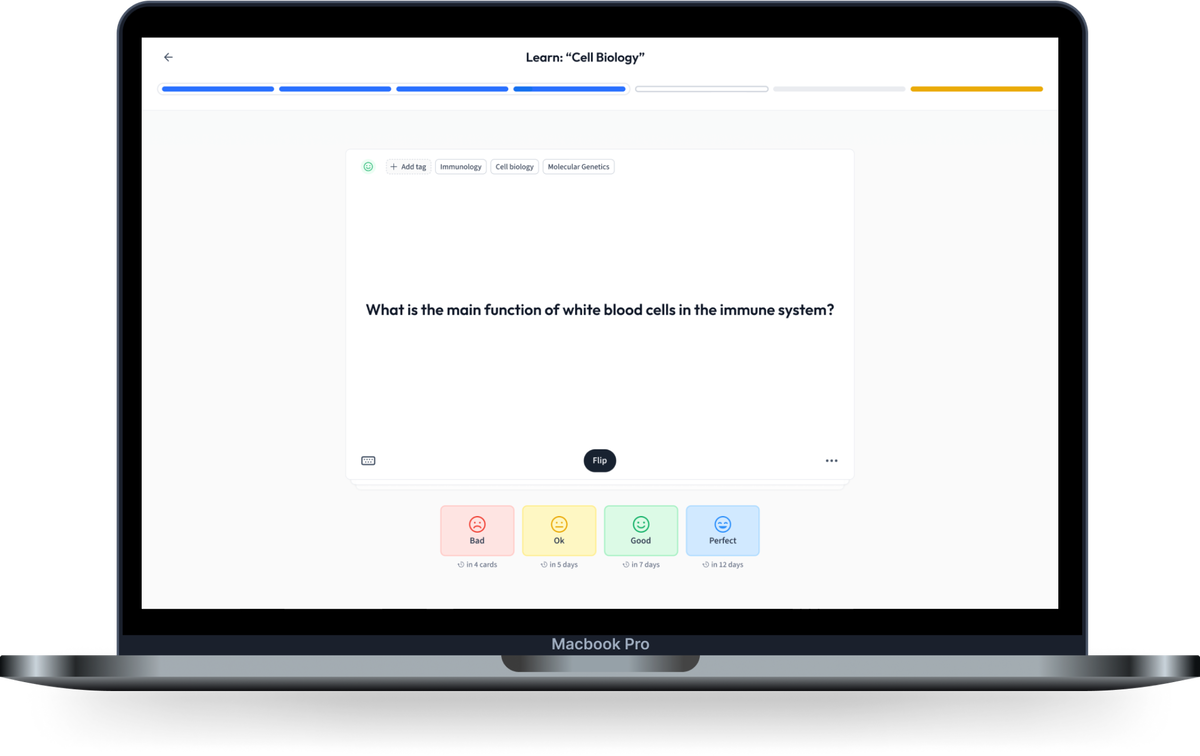
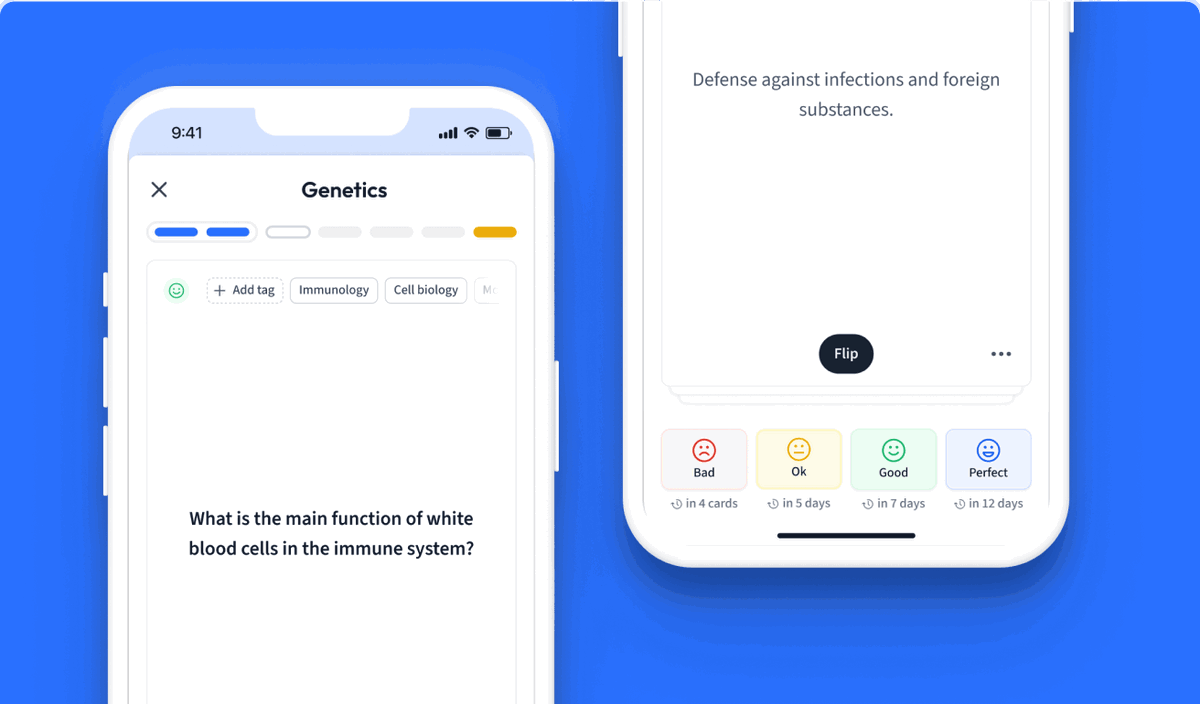
Learn with 15 Random Coil flashcards in the free StudySmarter app
Already have an account? Log in
Frequently Asked Questions about Random Coil
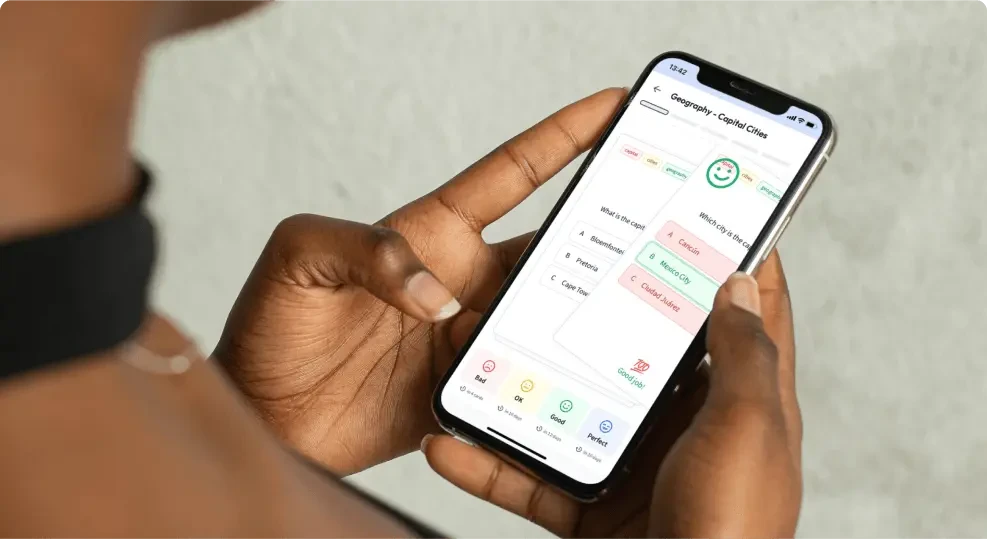
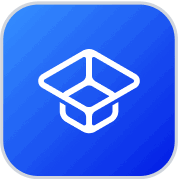
About StudySmarter
StudySmarter is a globally recognized educational technology company, offering a holistic learning platform designed for students of all ages and educational levels. Our platform provides learning support for a wide range of subjects, including STEM, Social Sciences, and Languages and also helps students to successfully master various tests and exams worldwide, such as GCSE, A Level, SAT, ACT, Abitur, and more. We offer an extensive library of learning materials, including interactive flashcards, comprehensive textbook solutions, and detailed explanations. The cutting-edge technology and tools we provide help students create their own learning materials. StudySmarter’s content is not only expert-verified but also regularly updated to ensure accuracy and relevance.
Learn more