Jump to a key chapter
Understanding Special Relativity: A Comprehensive Guide
In the exciting world of physics, the concept of Special Relativity plays a significant role. Developed by the renowned physicist Albert Einstein, it shook the foundations of classical physics and introduced groundbreaking ideas that significantly altered our understanding of time and space.
What is Special Relativity: Breaking Down Einstein's Revolutionary Concept
Special Relativity is a theory in physics that deals with the structure of spacetime. It describes how measurements of various physical phenomena vary for observers in different inertial frames of reference. This theory basically states that the laws of physics are the same in all inertial frames, and that the speed of light in a vacuum is the same for all observers, regardless of their motion or the source of light.
To understand it better, consider you're on a train moving at a constant speed. If you throw a ball up in the air, it will appear to follow a straight path up and down to you (an observer in the train). But to an observer standing still on the platform, the ball will follow a parabolic trajectory. This difference arises due to the different frames of reference of the two observers; yet, all physical laws (like gravity acting on the ball) were consistent in both frames.
The Core Elements of Einstein's Theory of Special Relativity
Special Relativity, as proposed by Einstein, is composed of two postulates, which lay the groundwork:
- The Principle of Relativity: The laws of physics are the same in all inertial frames of reference.
- The Constancy of the Speed of Light: Light in a vacuum travels at a constant speed, regardless of the motion of the source or the observer.
This theory forward, Einstein was able to arrive at some remarkable revelations, like the famous mass-energy equivalence, expressed as \(E=mc^2\), where \(E\) is energy, \(m\) is mass, and \(c\) is the speed of light. This equation suggests that mass and energy are interchangeable.
Achievements and Challenges of the Einstein Theory of Special Relativity
Achievements | Challenges |
Proposed a unified description of space and time | Relativity is non-intuitive and hard to visualize |
Proved the equivalence of mass and energy (\(E=mc^2\)) | Difficult mathematical aspects |
Provided a theoretical framework for the development of modern physics | Reconciling relativity with quantum mechanics |
The implications of Special Relativity are vast and far-reaching. From GPS systems that require corrections for time dilation to the principles behind nuclear energy, Einstein's revolutionary theory has provided the basis for numerous technological and scientific advancements.
Comparing Special Relativity and General Relativity: Discerning the Differences
When delving deeper into the realm of relativity, you'll begin to distinguish between two key constructs - Special Relativity and General Relativity. While interconnected, these theories maintain notable distinctions in scope and implications.
Special Relativity vs General Relativity: Contrasts under the Spotlight
Special Relativity, as previously defined, deals with objects moving at any speed, as long as it's constant. However, General Relativity, essentially an expansion of Special Relativity, factors in acceleration and the effects of gravitation. It provides a comprehensive explanation for the force of gravity and its impact on space and time.
While the laws of physics apply identically in all inertial frames in Special Relativity, General Relativity extends these principles to non-inertial (accelerating) frames as well. A critical outcome of this is the prediction of gravity as a curvature of spacetime caused by mass and energy.
Discovering the Unique Elements in Special Relativity Equations
When you assess the mathematics of Special Relativity, you'll encounter unique elements not found in classical physics. The Lorentz Transformation equations, named after Hendrik Lorentz, enable you to switch between two inertial frames of reference. Expressed as:
\[ x' = \gamma (x - ut) \] \[ t' = \gamma (t - ux/c^2) \]Where, \(x\), \(t\) are the coordinates in one reference frame, and \(x'\), \(t'\) are the coordinates in the other. \(u\) is the relative velocity between the frames and \(\gamma\) (Lorentz factor) is defined by the equation \(\gamma = 1/\sqrt{1 - u^2/c^2}\).
In an example scenario, imagine an observer moving at a constant speed in a spaceship, where a beam of light is travelling from the rear of the spaceship to the front. The Lorentz Transformation equations could be used to calculate the perception of that light beam's path for a stationary observer outside the spaceship.
Factors That Make General Relativity Distinct from Special Relativity
In General Relativity, gravitation plays a pivotal role. This theory introduces the monumental notion of space and time curving in the presence of mass and energy. Mathematicians use a set of ten equations, known as the Einstein Field Equations, to describe this interspace.
\[ G_{\mu\nu} = 8\pi G(T_{\mu\nu}-\frac{1}{2}g_{\mu\nu}T^{\alpha}_{\ \alpha}) \]Where \(G_{\mu\nu}\) represents the curvature of space-time, \(T_{\mu\nu}\) is the stress-energy tensor that reports the distribution of matter and energy, and \(g_{\mu\nu}\) is the metric tensor that encodes the geometry of spacetime.
One highlight of General Relativity is the phenomenon of Gravitational Lensing. In this effect, light rays from a distant source bend when they pass through the gravitational field of an intervening object, causing the source to appear distorted or magnified to an observer.
Profound consequences of General Relativity are expansions of the universe and black holes - massive objects with gravitational pull so strong even light can't escape. These are absent in the Special theory, demonstrating the scope of the General theory of Relativity.
Probing into Key Aspects of Special Relativity - Time Dilation, Length Contraction, and Simultaneity
Special Relativity harbours some truly captivating ideas. Among its several unique aspects, three features stand out as particularly significant: time dilation, length contraction, and simultaneity. These phenomena radically challenge our everyday perceptions of time and space.
Special Relativity Simultaneity and Time Dilation: A Journey Through Time Altered
The intriguing realms of simultaneity and time dilation offer a novel perspective on the fabric of space-time. Each appears to contradict familiar notions, presenting a world where time can slow down and simultaneous events vary from one observer to another.
The Concept of Simultaneity in Special Relativity: Alignments in Perspective
Simultaneity in Special Relativity is the notion that the concept of events happening at the same time is not absolute but is relative to the observer. In other words, two events that appear simultaneous to an observer in one frame of reference may not appear simultaneous to an observer in a different frame.
Consider two entities, Alice and Bob, moving relative to each other. If two flashes occur at the same location for Alice and she declares them simultaneous, Bob, travelling relative to Alice, would not agree. For him, due to the finite speed of light and his movement, he would observe them at different times, thus breaking the simultaneity for him.
Unraveling the Paradox of Time Dilation in Special Relativity
Time dilation is a fascinating outcome of Special Relativity, which proposes that a moving clock will appear to run slower than a stationary one. This isn't simply a perceptual trick; it's a real, physical phenomenon.
For illustration, consider a spaceship traveling near the speed of light. A passenger in the spaceship might measure the time to travel to a nearby star as one year, while to a stationary observer, the journey might appear to take many decades. This difference in elapsed time is a direct consequence of Time Dilation, a reality that seems counterintuitive but is firmly grounded in the laws of Special Relativity.
The derivation of time dilation is based on the invariance of the spacetime interval. The math behind it involves using the Lorentz transformation and results in the time dilation formula: \( \Delta t' = \gamma \Delta t \), where \(\Delta t\) and \(\Delta t'\) are the time intervals as measured in the stationary and moving frames, respectively, and \(\gamma\) is the Lorentz factor.
Special Relativity Length Contraction: A New Dimension of Space
Length contraction is another stunning revelation from Special Relativity. This phenomenon, akin to time dilation, profoundly transforms our understanding of spatial dimensions.
Length Contraction in Special Relativity: An Overview
Length contraction in Special Relativity is the phenomenon where an object in motion seems shorter along the direction of motion as observed from a stationary frame of reference when compared to its proper length (length as measured in the object's rest frame).
This physical shortening isn't due to any mechanical compression but is a fundamental aspect of how space and time are intertwined in the theory of Special Relativity. Again, a mathematical consequence of the invariance of the spacetime interval, the formula for length contraction can be demonstrated as: \(L = L_0 / \gamma \), where \(L\) is the contracted length, \(L_0\) is the object’s proper length, and \(\gamma\) is the Lorentz factor.
The Phenomenon of Length Contraction: What Makes It Integral to Special Relativity
Along with time dilation, length contraction forms the bedrock of Special Relativity. It's not merely a bizarre side effect but a central feature that ensures the speed of light remains invariant for all observers. It further introduces the critical concept that space and time are inextricably linked, creating the four-dimensional fabric of the universe - spacetime.
Time dilation and length contraction may sound paradoxical because they violate our ordinary experience. However, since these effects are negligible at velocities much less than the speed of light, they aren’t observed in our day-to-day life. They only become apparent at speeds approaching the speed of light, which, in our current technological state, is predominantly limited to the realm of subatomic particles and cosmic observations.
The Role of Experiments in Validating Special Relativity
As in all scientific disciplines, experimental verification plays a vital role in physics. Theories, no matter how mathematically beautiful or logically sound, hold little weight without empirical confirmation. Special Relativity, despite its abstract and counterintuitive ideas, also had to face the rigours of experimental validation. One such key experiment that confirmed the validity of the principles of Special Relativity was the Michelson-Morley experiment, a hallmark in the history of physics.
Special Relativity: The Pivotal Michelson Morley Experiment
The Michelson-Morley experiment, conducted in the late 19th century, occupies a significant place not just in verifying Special Relativity, but in fundamentally shaping its formation. Designed to detect the 'ether wind', the perceived relative motion of Earth through the hypothetical luminiferous ether (the medium proposed to carry light waves), the experiment yielded results that directly fed into developing the theory of Special Relativity.
The Michelson Morley Experiment: A Breakthrough in Validating Special Relativity
The Michelson-Morley experiment was an intricate interference experiment conducted by Albert A. Michelson and Edward W. Morley. It utilised a device called the Michelson interferometer, capable of detecting tiny shifts in the interference patterns of light waves. The experiment aimed to measure the slight changes in the speed of light due to Earth's supposed motion through the luminiferous ether.
Think of the set-up like rowing a boat downstream then upstream on a moving river. One would expect the downstream (with the current) trip to be faster than the upstream trip (against the current). By an analogous logic, if light were moving through ether (the hypothetical 'river'), then the speed of light should differ based on the direction of travel. This speed change should then show up as a shift in interference patterns.
The experiment, however, found no such shift. The null result was a striking revelation - there wasn't any preferred direction of light travel. In essence, instead of affirming the prevalent ether theory, the Michelson-Morley experiment negated it, providing a compelling piece of the puzzle that later culminated in Einstein's Special Theory of Relativity.
The Impact and Understanding Gained from the Michelson Morley Experiment
The Michelson-Morley experiment, despite being a 'failed experiment' in the sense of disproving the hypothesis it was designed to test, provided insight into a deeper truth - one that challenged the foundations of classical physics.
Its null result didn't just refute the ether theory, it redefined the understanding of space and time. By indicating that the speed of light is invariant, independent of the motion of the source or the observer, it lent empirical support to the second postulate of Special Relativity. Its impact wasn't immediate, but its implications were far-reaching.
Often called the most famous failed experiment, the Michelson-Morley experiment is celebrated for its integrity in transforming a negative result into a profound scientific advancement. Its understanding wasn't just about refuting the existence of ether, but about opening doors for a new way of thinking about the fundamental nature of the universe.
The experimental underpinning of Special Relativity by the Michelson-Morley experiment underlines a critical aspect of scientific progress. It showcases how experimental results, whether in agreement or disagreement with prevailing theories, lead to a more accurate understanding of our universe by either confirming, refuting, or refining theoretical ideas.
Exploring Real-world Applications of Special Relativity
While the principles of Special Relativity may seem abstract and removed from our daily experiences, this groundbreaking theory actually has numerous applications that significantly impact our everyday lives. From enabling GPS technologies to enhancing our understanding of particle physics, it serves as a critical foundation across diverse facets of modern life.
Special Relativity Examples: From Theory to Practice
Delving deeper into the practical implications of Special Relativity can be quite eye-opening. It transforms this seemingly abstract physical theory into a tangible construct that we interact with on a regular basis. Here, we explore a few instances that demonstrate the principles of Special Relativity quite vividly.
Instances Demonstrating the Principles of Special Relativity
A compelling demonstration of Special Relativity principles is the lifetime of muons. Muons are subatomic particles created in Earth's upper atmosphere by cosmic rays. Without time dilation, these particles, decaying within microseconds, wouldn't make it to the ground. But due to their high speed (near light speed), time dilation comes into play and extends their lifespan from the viewpoint of an observer on Earth, enabling us to detect these particles at ground level. It's a real-life instance where we see Special Relativity's time dilation principle at work.
Another example of Special Relativity in action is found in the world of particle accelerators. These machines use electric fields to accelerate charged particles to near light speeds. Due to the effects of relativistic mass increase (an outcome of Special Relativity), the particles gain energy becoming more massive as they approach the speed of light. This principle is used in experiments at facilities like CERN's Large Hadron Collider to study fundamental aspects of our universe.
Practical Manifestations of Special Relativity Equations in Everyday Life
The principles of Special Relativity, while conceptually fascinating, are not just limited to theoretical or experimental physics; they also find substantial application in everyday technologies we rely on.
The Global Positioning System (GPS), a technology integral to navigation and precise timing, provides one of the most direct applications of Special Relativity. The GPS network comprises satellites orbiting Earth, constantly sending time-stamped information to receivers on the ground. Due to the high relative speeds of the satellites (which gives rise to time dilation) and their distance from Earth's surface (where gravity has a different strength, affecting time as per General Relativity), Special and General Relativity corrections are essential to providing accurate positioning data. Without accounting for these effects, GPS coordinates would go off by several kilometres within just a day!
Interestingly, Special Relativity also finds a crucial application in the healthcare industry. Particle accelerators used in cancer treatments, such as proton therapy, rely on Special Relativity. These machines accelerate protons close to the speed of light, causing them to gain mass due to the relativistic mass increase. These high-energy protons can then be targeted with extreme precision to destroy cancerous cells, minimising damage to surrounding healthy tissue.
Thus, from guiding us to our destinations to advancing medical treatments and cosmological understanding, Special Relativity is much more than an abstract concept - it's an integral part of our technologically advanced world.
Special Relativity - Key takeaways
- Special Relativity is a theory that deals with objects moving at a constant speed and provides a unified description of space and time.
- Special Relativity is distinguished from General Relativity through its regard for objects moving at any speed, as long as it's constant, whereas General Relativity factors in acceleration and the effects of gravitation.
- A prominent concept in Special Relativity is the Lorentz Transformation equations, which enable switching between two inertial frames of reference.
- Key aspects of Special Relativity include time dilation (where a moving clock will appear to run slower than a stationary one), length contraction (objects in motion seem shorter along the direction of motion as observed from a stationary frame of reference) and simultaneity (events happening at the same time is not an absolute concept but relative to an observer's frame of reference).
- The Michelson-Morley experiment, which found that the speed of light is invariant, provided empirical support for the theory of Special Relativity.
Learn faster with the 20 flashcards about Special Relativity
Sign up for free to gain access to all our flashcards.
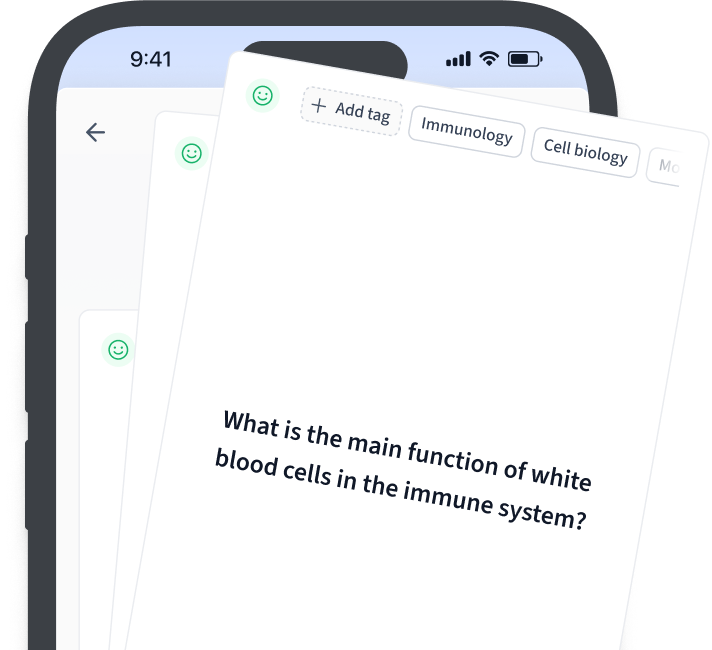
Frequently Asked Questions about Special Relativity
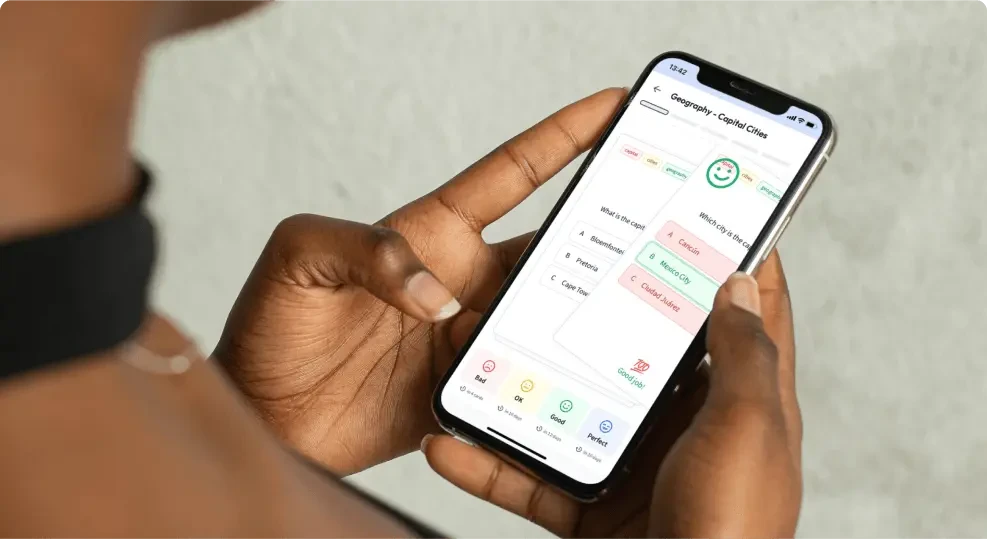
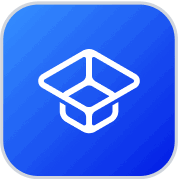
About StudySmarter
StudySmarter is a globally recognized educational technology company, offering a holistic learning platform designed for students of all ages and educational levels. Our platform provides learning support for a wide range of subjects, including STEM, Social Sciences, and Languages and also helps students to successfully master various tests and exams worldwide, such as GCSE, A Level, SAT, ACT, Abitur, and more. We offer an extensive library of learning materials, including interactive flashcards, comprehensive textbook solutions, and detailed explanations. The cutting-edge technology and tools we provide help students create their own learning materials. StudySmarter’s content is not only expert-verified but also regularly updated to ensure accuracy and relevance.
Learn more