Jump to a key chapter
Understanding the Concept of Coded Aperture
Delving into the world of Physics, there are numerous concepts that you'll come across, one of which is the intriguing idea of a coded aperture. This is a concept that is primarily utilised in the field of high-energy astronomy and plays a fundamental role in enhancing the clarity and resolution of images.
What is a Coded Aperture?
Before you dive deeper into the mechanisms of a coded aperture, it's crucial to grasp a basic understanding of what it entails. A coded aperture is essentially a type of imaging technique that is used to enhance image resolution, particularly in the process of studying celestial bodies.
By definition, a coded aperture is a type of telescope aperture that utilises a patterned opening to enable the telescope to demonstrate a wider field of view as compared to a traditional pinhole telescope.
It's typically used in contexts where lens-based imaging is impractical or impossible. This includes fields such as X-ray and gamma-ray astronomy where the astronomical objects to be observed are so far away that traditional optical telescopes struggle to capture a clear image.
For instance, in X-ray astronomy, X-rays from distant celestial bodies can't be focused by traditional lenses because they tend to get absorbed or refracted. Here, using a coded aperture helps as it allows for the creation of a shadowgram on the detector plane, which can then be decoded to form the original image.
Essential Elements of a Coded Aperture
Now that you've understood what a coded aperture is, let's delve deeper into its core elements. A coded aperture system mainly consists of an aperture pattern, a detector plane, and a decoding algorithm.
- Aperture Pattern: The aperture pattern, as the name suggests, refers to the pattern in which the aperture is coded. This pattern is specifically designed to allow multiple paths for the light to reach the detector, thus enhancing its resolution.
- Detector Plane: The detector plane is where the coded shadowgram is formed, which is a distorted image of the light source observed through the coded aperture pattern.
- Decoding Algorithm: The decoding algorithm is a mathematical procedure which is used to decode or deconvolve the shadowgram to obtain a clear image of the light source.
These elements work together to ensure the effective functioning of a coded aperture. The aperture pattern allows for more photon paths to reach the detector plane, the detector plane records the resulting shadowgram, and the decoding algorithm then deciphers this shadowgram to reconstruct the image of the light source.
For example, in X-ray astronomy, the coded aperture pattern is usually a grid or a pattern of holes. The X-ray photons pass through these holes, creating a shadowgram on the detector plane. Then, the decoding algorithm uses the shape and intensity of the shadowgram to determine the position and brightness of the X-ray source.
Some standard coded aperture patterns used in astronomy include Uniformly Redundant Arrays (URA), Non-Uniformly Redundant Arrays (NURA), and Modified Uniformly Redundant Arrays (MURA). These array patterns have certain advantages in specific contexts and greatly enhance the efficiency of the imaging process.
Delving into Coded Aperture Imaging
When talking about coded aperture imaging, it's essentially a technique used in situations where lens-based imaging is impractical, such as in X-ray and gamma-ray imaging. With the help of specially crafted aperture patterns, you can receive multiple, overlapping images of a light source on a detector plane, which, after decoding, provides the final image.
Basics of Coded Aperture Imaging
Taking a closer look at coded aperture imaging, there are a few fundamental principles that you need to understand. This imaging technique leverages the process of diffraction and interference to create an image from the patterned light detected on the detector plane.
Diffraction: It refers to the phenomenon where waves, including light waves, spread as they pass through an aperture or around an obstacle.
Interference: It's the interaction of waves, resulting in a new wave pattern. For instance, several overlapping images can create an interference pattern on the detector plane in the context of coded aperture imaging.
Crucial to the process of coded aperture imaging is the understanding of how the aperture pattern is structured. The specially crafted aperture pattern mimics the function of a lens by letting light from different directions fall onto different parts of the detector plane. The geometry of the aperture pattern is usually designed to feature a highly autocorrelative function, which simply means that the pattern is repeated at different scales, allowing it to capture more detailed information from the light source.
One of the main equations central to coded aperture imaging is the autocorrelation function of the aperture pattern, given by \[ R(m,n) = \sum_{i=0}^{i=S-1} \sum_{j=0}^{J=S-1} P(i,j)P(i+m,j+n) \] where \( R(m,n) \) is the autocorrelation function, \( P(i,j) \) refers to the aperture grid, \( S \) is the size of the aperture grid, and \( (m, n) \) are the shifts in the aperture pattern.Application of Coded Aperture Imaging
Coded aperture imaging isn't just restricted to the field of astronomy. Its utility extends to various other sectors as well. For instance, in medical imaging, coded apertures have been successfully used in nuclear medicine for gamma-ray imaging.
In nuclear medicine, capturing images of gamma rays emitted from a patient's body helps in the diagnosis of several diseases. Traditional gamma cameras often lack in providing high-resolution images. However, implementing coded aperture imaging, thanks to its ability to handle high-energy photons like gamma rays, can significantly enhance the resolution and thus lead to more accurate diagnosing.
Exploring the Coded Aperture Camera
A coded aperture camera is essentially a camera that uses a coded aperture instead of a conventional lens. This coded aperture plays the critical role of manipulating the incoming light in a way that it improves the camera's resolution and depth of field.
There are several types of coded apertures that a coded aperture camera can implement. For example:
- Fresnel Zone Plate: It's a circular type of aperture that has stages of alternating transparency and opacity. It behaves like a lens by focusing light onto a single point through diffraction.
- MURA (Modified Uniformly Redundant Array): As an incredibly efficient aperture design for coded aperture cameras, it is known to produce images with an enhanced signal-to-noise ratio.
- Poisson Disk Pattern: It's a random distribution of opaque and transparent points that is highly desirable for its balance between resolution and noise reduction.
Usage of Coded aperture camera in Physics
While coded aperture cameras find their significant application in astronomical observations, you can also find their usefulness in different sectors within physics. One such notable usage is in the field of quantum mechanics.
Consider the famous double slit experiment used to demonstrate the principle of wave-particle duality. Here, a coded aperture camera with an appropriately designed aperture can add more slits to the double slit experiment. This adjustment allows the experiment to capture more interference patterns, thus enabling more detailed observations of the quantum mechanical phenomena.
In nuclear physics, a coded aperture camera can help provide more accurate images of radioactive decay and nuclear reactions. By being capable of handling high-energy photons emitted during these processes, it provides detailed images of the events, resulting in a deeper investigation and understanding of the subject.
Finally, in particle physics, a coded aperture camera can capture high-energy particles like gamma rays and neutrinos directly, providing precise images that enhance our understanding of these particles and their properties.
Overview of Coded Aperture Snapshot Spectral Imaging
Coded Aperture Snapshot Spectral Imaging (CASSI) is a revolutionary imaging technology that enables the acquisition of spectral and spatial data in a snapshot, meaning it captures the complete three-dimensional data cube in one shot. The technique utilises the coded aperture concept to detect both the spatial and spectral information of the light source, thereby overcoming one of the major challenges observed in other spectral imaging techniques, which typically require mechanical scanning and hence, longer imaging times.
Introduction to Coded Aperture Snapshot Spectral Imaging
Coded Aperture Snapshot Spectral Imaging (CASSI) is a powerful tool that strives to provide a comprehensive understanding of the object under observation by capturing both the spatial and spectral information simultaneously. Here, the term spectral information refers to identifying the various wavelengths of light that an object absorbs, reflects or emits and spatial information refers to the physical location of these spectral characteristics.
Spatial Information: It takes into account the physical location or the spatial distribution of the object's properties. In the context of imaging, it usually refers to the X-Y coordinates of the pixels that make up the object's image.
Spectral Information: It refers to the different wavelengths that are either emitted, reflected, or absorbed by the object. Spectral information can give significant insights into the object's characteristics, such as its composition, temperature, chemical constituents, and more.
In a CASSI system, a two-dimensional (2D) coded aperture or mask is used, which is spectrally dispersed onto the detector plane. This process of dispersion at the detector plane essentially encodes both the spatial and spectral information of the object. This encoded information is then passed through a mathematical transformation for decoding, turning this encoded information into a three-dimensional (3D) data cube containing the different spectral signatures at various spatial locations of the object.
In the decoding phase, the process typically involves solving an inverse problem to recover the object's image from the captured data. This can be done using various sophisticated image reconstruction algorithms with mathematical models such as the \( l\_1 \)-norm minimisation for sparse representation of signals.
Role of Coded Aperture Snapshot Spectral Imaging in Physics
In the sphere of Physics, Coded Aperture Snapshot Spectral Imaging finds extensive applications. As it can simultaneously capture spatial and spectral information, it provides more comprehensive data representation. This advanced technique is particularly beneficial in the realm of spectroscopy and material analysis, and is extensively used in labs and industries alike.
For example, in spectroscopy, the spectral signatures captured by CASSI can help identify various elements or compounds in a sample based on their distinct absorption, reflection, and emission characteristics. This can be applicable in determining the composition of celestial bodies via non-invasive methods in astronomy, detecting impurities or inclusions in materials in material science, or analysing the composition of solutions in chemistry and pharmaceutical industries.
CASSI also contributes significantly to hyperspectral imaging, an advanced imaging method that gathers and processes information from across the electromagnetic spectrum. Thereby, it plays a crucial role in domains like remote sensing and environmental studies, helping to identify materials, detect processes, and find objects. Its contribution to precision agriculture is particularly noteworthy, helping in assessing field variance, disease detection, and yield estimation.
Beyond these, CASSI finds its use in medical imaging, helping in early detection and diagnosis of diseases by visualising changes at the molecular level, extending its benefits to clinical diagnostics and treatment monitoring. From scanning the atmosphere for pollution monitoring to art and heritage science for pigment identification and artwork analysis, the role of Coded Aperture Snapshot Spectral Imaging is far-reaching, truly enhancing our ability to observe and perceive our world.
Adaptive Coded Aperture Imaging and Non-Imaging Sensors
Adaptive Coded Aperture Imaging (ACAI) is a remarkable advancement in the field of imaging technology that brings about a new form of flexibility and adaptability to coded aperture imaging systems. On the other hand, non-imaging sensors are devices not primarily designed to form an image but are capable of collecting other types of data, such as temperature, pressure, chemical composition, and others. Both ACAI and non-imaging sensors have brought about significant contributions in enhancing our ability to acquire comprehensive and varied data from our environment.
Meaning and Functionality of Adaptive Coded Aperture Imaging
Adaptive Coded Aperture Imaging (ACAI) brings together the principles of traditional coded aperture systems and adaptivity. This means that adaptive coded apertures can alter their patterns in real time to adapt to the scene's specific requirements, thereby optimising the image quality. An ACAI system can change the coverage, density, and distributions of the diffraction patterns by modifying its aperture pattern - a feature that traditional coded aperture systems lack.
Adaptivity: It refers to the ability of a system to modify its parameters and behaviour dynamically in response to changes in the input or environment.
The functionality of an ACAI system is not drastically different from a traditional coded aperture imaging system. However, the primary distinction lies in how the ACAI system can promptly respond to changing imaging conditions and requirements. The ACAI system captures multiple images through different aperture patterns and then combines them using advanced algorithms to reconstruct a high-resolution image of the scene.
Decoding the data collected by the ACAI requires a robust reconstruction algorithm. However, the algorithm's complexity is increased due to the additional factor of changing coded aperture patterns. Hence, the mathematically advanced reconstruction procedures need to consider this dynamism. Its reconstruction algorithm can be represented as:
\[ X_{reconstructed} = F^{-1}( \hat{H} \cdot F( Y / \hat{H} ) ) \] where \( X_{reconstructed} \) is the reconstructed image, \( F \) is the Fourier transform, \( Y \) is the captured data, \( \hat{H} \) represents the combined response of the aperture patterns and the imaging system.Understanding the Non-Imaging Sensors
Non-imaging sensors are those types of sensors that aren't primarily meant to form an image. Rather, they are designed to capture different types of data to give extensive information about the object or environment. From temperature sensors, pressure sensors, motion detectors to chemical sensors - each of these provides vital information that might be missed by an imaging system.
Non-imaging sensors work on the basis that's fundamentally different from imaging sensors, as it's not the formation of an image that's at stake here. Instead, these sensors focus on detecting and measuring the specific parameter they're designed for. For instance, a temperature sensor works primarily based on changes in its physical characteristics due to temperature alterations.
Let's consider an example - a thermistor, a type of temperature sensor. These typically change their resistance with alterations in temperature. The change in resistance can be calculated using the formula:
\[ R_T = R_0 e^{B(\frac{1}{T}-\frac{1}{T_0})} \] where \( R_T \) is the resistance at temperature \( T \), \( R_0 \) is the resistance at the reference temperature \( T_0 \), \( B \) is the material constant, and \( e \) is the base of natural logarithm.Advantages of Adaptive Coded Aperture Techniques
The advent of Adaptive Coded Aperture Techniques heralds a new era in the field of imaging sciences. The capability to modify the aperture pattern to optimise image quality gives ACAI a clear advantage over its traditional counterparts in many facets. Here are some of the key advantages:
- Flexibility: ACAI technologies offer the flexibility to adapt the code patterns according to the imaging scenario. It allows them to handle diverse imaging conditions dynamically, which is not achievable with traditional systems having fixed aperture patterns.
- Optimised Image Quality: By adjusting the pattern and density of apertures to the specific needs of the scene being imaged, ACAI systems can significantly contribute to enhanced image resolution, reduced noise levels, and improved signal-to-noise ratio, leading to overall better image quality.
- Efficient Data Acquisition: The adaptive nature of ACAI ensures efficient data acquisition by focusing on relevant spatial or spectral regions based on the scene's demand, thereby reducing redundant data.
- Enhanced System Performance: ACAI systems, with their adaptive nature, can improve system performance through increased operational speed, energy efficiency, and effective resource management as it focuses only on relevant aspects.
Thus, the implementation of Adaptive Coded Aperture techniques brings about a significant improvement in the imaging quality and functionality, fostering advancements in various fields, including Physics, which heavily rely on capturing comprehensive and accurate data about the environment and phenomena.
The Physics of Coded Aperture
By now, you'll have grasped the concept of coded apertures, and how they're changing the face of imaging technology. But, have you ever wondered about the physics that makes this technology possible? If yes, you're in the right place! Studying the physics behind coded aperture isn't just about formulae and equations. In reality, it's an interplay of exciting ideas that have helped create an influential technology in imaging sciences.
Coded Aperture Explained
Coded Aperture is a breakthrough in imaging technology that allows experts to capture comprehensive data of a scene even in challenging conditions like low light, extreme brightness, or turbidity. It emerged from the necessity to improve imaging capabilities, especially in sectors like deep-space exploration, medical imaging, and high-energy spectral imaging. But how does it work? It's all about Physics!
Coded Aperture: It is a type of imaging system that uses a patterned aperture to capture detailed data of a scene or object. This aperture pattern codes the incoming light, which can be subsequently decoded to reveal a high-resolution image.
Traditional imaging systems are restricted by a single aperture that lets light pass through it to the sensor. This limitation hampers the resolution and clarity, as the system can only use the light that passes through the aperture. However, coded aperture systems are different. They have multiple apertures in a known pattern that collects spatial and spectral data about an object or scene. These apertures together form a coded mask, and the patterns in this mask encode the incoming light rays.
Consider a simple pinhole camera, where the single aperture - the pinhole, carries the entire burden of capturing the image detail. Now imagine a camera with numerous pinholes, each adding to the image's detail and quality. That's precisely what a coded aperture offers!
Each aperture in the coded mask emits a pattern onto the detector or sensor. These patterns overlap and form a complicated, mixed pattern. The resultant pattern is the coded image, where the incoming light rays are marked by aperture patterns. Incredible, isn't it?
Decoding the Coded Aperture Technique
Decoding the coded aperture is an integral part of the coded aperture technology. The mixed pattern received by the detector is decoded using computational algorithms, thereby revealing a high-resolution image. The decoding process is essentially an inversion of the encoding process carried out by the aperture patterns.
Complex mathematics and computation underpin this decoding mechanism. Each aperture contributes to multiple points on the detector, and similarly, each point on the detector receives light from multiple apertures. As a consequence, the detected signal at a particular point includes the sum of signals from multiple apertures.
To understand this, think of multiple torchlights shining onto a screen at overlapping points. The brightness detected at the overlapped point is the sum of the brightness from each torchlight. Similarly, in a coded aperture system, each point on the detector receives light from different apertures. Hence, every point on the detector has a unique pattern associated with it, which is the sum of patterns associated with individual apertures.
The decoding algorithm follows one basic principle: if the coded mask and the detector are known, then theoretically, the image can be reconstructed. The decoding process mathematically unscrambles these overlapping patterns using advanced numerical techniques to reveal the source image.
Impact of Physics knowledge on Coded Aperture
Physics plays a vital role in understanding and implementing coded aperture technology. The principles of wave theory, diffraction, quantum mechanics, and optics find direct application in the technique of coded aperture. Concepts like interference and superposition of waves are at the core of the coding and decoding mechanisms of a coded aperture system.
Moreover, the mathematical models and computational algorithms used in decoding the image in a coded aperture are derived from physics principles. Without a comprehensive understanding of these principles, it would be difficult, probably impossible, to design and develop an efficient coded aperture system.
Unravelling the Physics behind Coded Aperture Technique
The foundation of coded aperture technology lies in the basic principles of light propagation and wave theory. When light encounters the aperture pattern, it behaves according to Huygens' principle, which suggests that every point on a wavefront is a source of light waves. Hence, when light interacts with the aperture pattern, each point on the aperture becomes a secondary source of light, illuminating the detector. The overlapping of these patterns due to superposition forms the coded image on the detector.
The principles of Physics shape the construction and placement of coded aperture masks, too. The coded masks' patterns have a direct influence on the quality of the resulting image, with some patterns offering better signal-to-noise ratio and resolution than the others.
An important physical principle in the decoding process is the Fourier Transform. This mathematical technique, which has roots in wave analysis, is used to switch from one domain (usually time or space) to another (frequency). It plays a vital role in unravelling the entangled signals received from different apertures and is a crucial part of the image reconstruction algorithm in a coded aperture system.
Remember when we mentioned that each point on the detector receives light from different apertures? Well, this is where Fourier Transform comes into play. It mathematically isolates these overlapping signals and presents them in a form that allows us to see the contribution of each aperture, thereby aiding in the decoding process.
Without these principles of Physics and their insightful application, the coded aperture technique would still be an unexplored area in imaging sciences. Physics not only enabled the advent of coded aperture technology but also continues to influence its enhancements and advancements. Fascinating, isn’t it?
Coded Aperture - Key takeaways
- Coded aperture imaging is an imaging technique used to enhance resolution, particularly in the field of nuclear medicine and astronomy.
- A coded aperture camera uses a coded aperture instead of a conventional lens to manipulate the incoming light and enhance the camera's resolution and depth of field.
- Types of coded apertures include the Fresnel Zone Plate, MURA (Modified Uniformly Redundant Array), and the Poisson Disk Pattern, each offering different benefits in image resolution and noise reduction.
- Coded Aperture Snapshot Spectral Imaging (CASSI) is an imaging technology that captures both spatial and spectral data in a single snapshot, overcoming challenges faced by other spectral imaging techniques that require mechanical scanning.
- Adaptive Coded Aperture Imaging (ACAI) allows for real-time adaptation of the aperture patterns to optimize image quality in varying imaging conditions. The changing aperture patterns contribute to the complexity of the reconstruction algorithms necessary to decode the image data.
- Non-imaging sensors are devices not intended to form an image but collect other types of data such as temperature, pressure, and chemical composition.
- The benefits of Adaptive Coded Aperture Techniques include flexibility, optimised image quality, efficient data acquisition, and enhanced system performance.
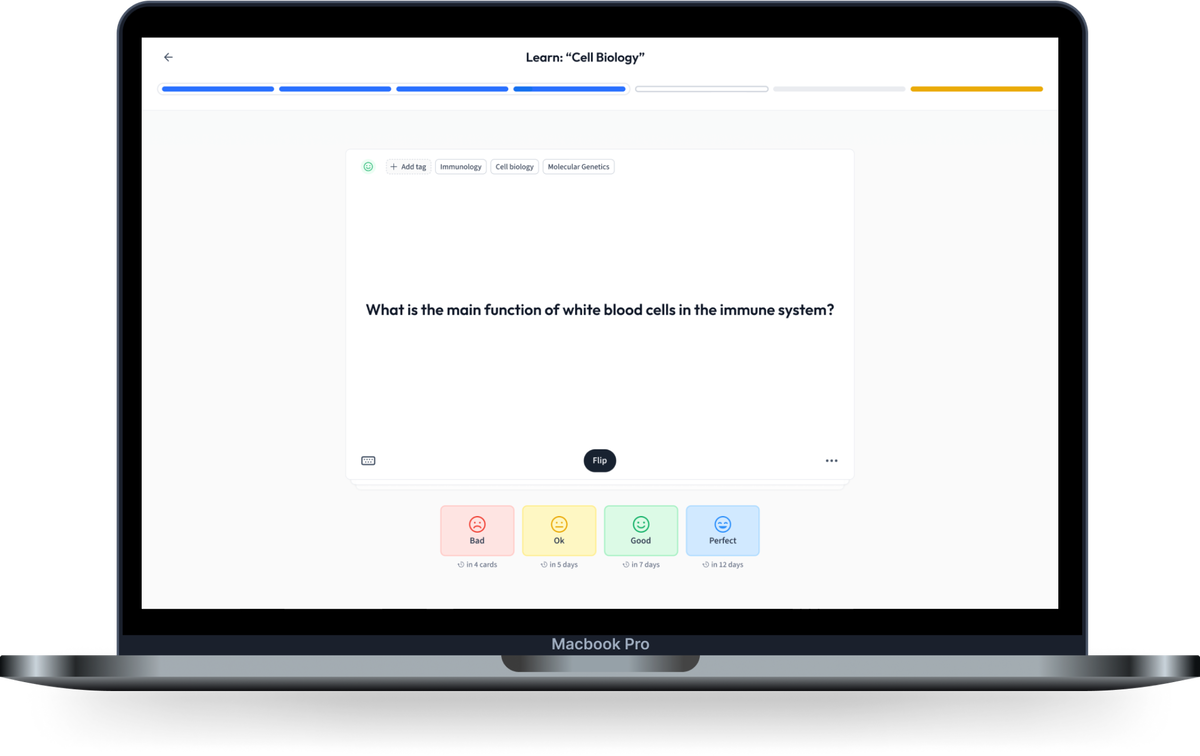
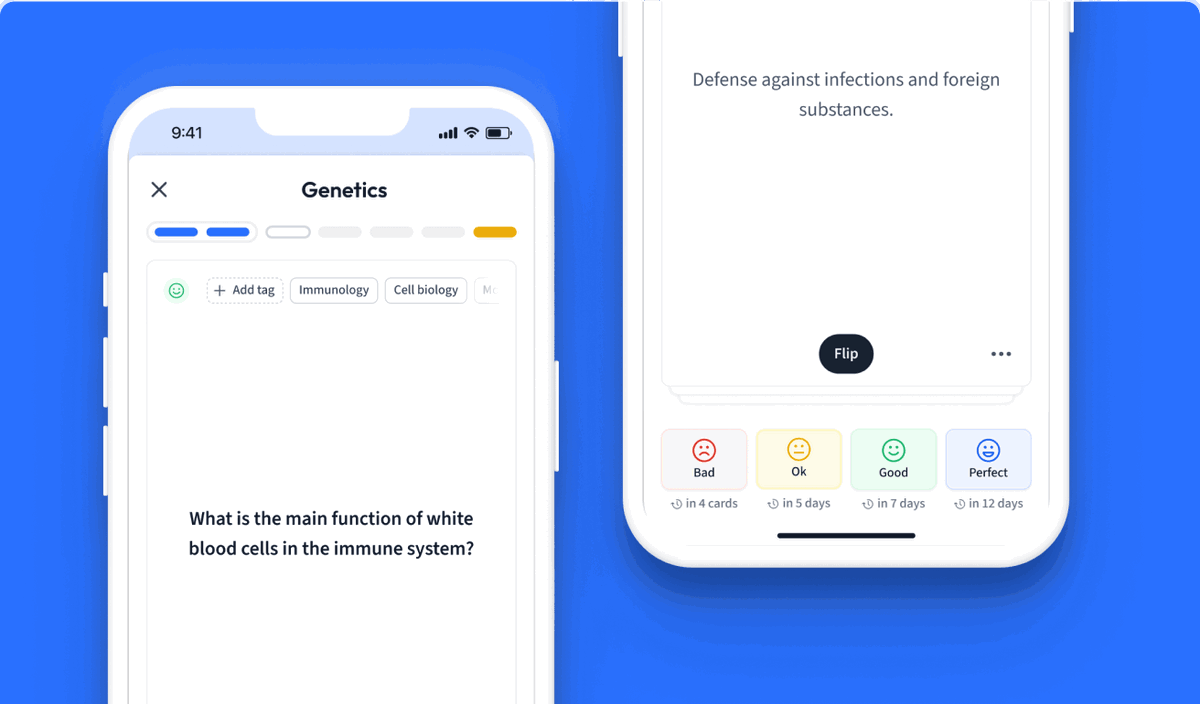
Learn with 15 Coded Aperture flashcards in the free StudySmarter app
Already have an account? Log in
Frequently Asked Questions about Coded Aperture
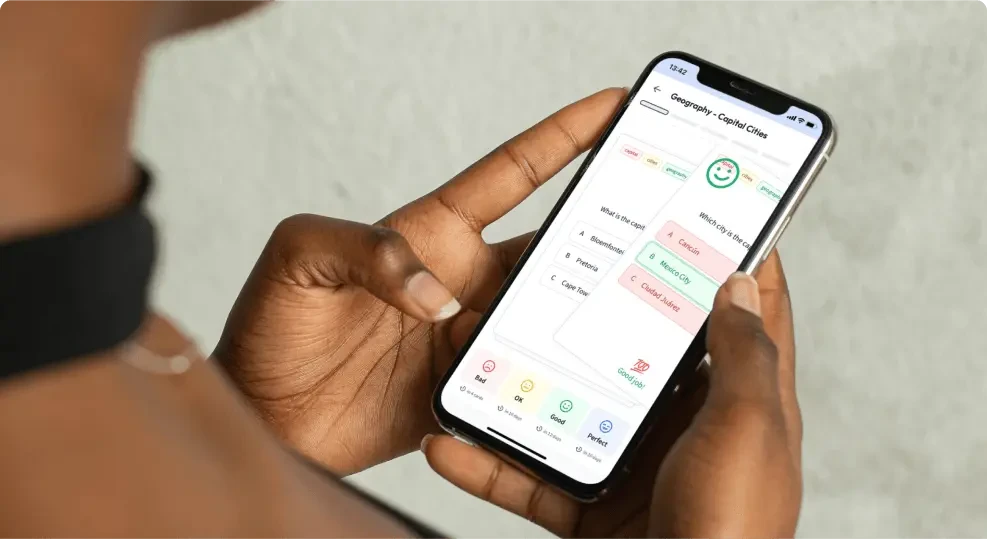
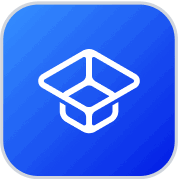
About StudySmarter
StudySmarter is a globally recognized educational technology company, offering a holistic learning platform designed for students of all ages and educational levels. Our platform provides learning support for a wide range of subjects, including STEM, Social Sciences, and Languages and also helps students to successfully master various tests and exams worldwide, such as GCSE, A Level, SAT, ACT, Abitur, and more. We offer an extensive library of learning materials, including interactive flashcards, comprehensive textbook solutions, and detailed explanations. The cutting-edge technology and tools we provide help students create their own learning materials. StudySmarter’s content is not only expert-verified but also regularly updated to ensure accuracy and relevance.
Learn more