Jump to a key chapter
Magnetoencephalography Overview
Magnetoencephalography (MEG) is a non-invasive imaging technique that measures the magnetic fields produced by neural activity in the brain. This method offers high temporal resolution, making it a valuable tool in both research and clinical settings.
What is Magnetoencephalography?
Magnetoencephalography (MEG): A brain imaging technique that detects the magnetic fields generated by neuronal activity. It provides high temporal resolution and is used to map brain functions.
MEG works by measuring the magnetic fields produced by electrical currents in the brain. These measurements are captured by extremely sensitive devices known as Superconducting Quantum Interference Devices (SQUIDs).MEG is unique because it measures magnetic fields directly, rather than inferring brain activity indirectly. This direct monitoring allows for greater precision in timing and localization of brain activity.
MEG is often coupled with other imaging techniques like MRI to get both the spatial resolution of structural imaging and the temporal resolution of functional imaging.
To accurately capture the brain's magnetic fields, an MEG machine needs to be housed in a magnetically shielded room. This room blocks external magnetic interference, ensuring the sensitivity of the measurements. The ultra-sensitive SQUID sensors are cooled to cryogenic temperatures to maintain their superconducting properties. This technological setup makes MEG one of the most sophisticated, albeit expensive, neuroimaging techniques available.
Applications of Magnetoencephalography
MEG has a wide range of applications in neuroscience and medicine. Some key application areas include:
- Mapping Brain Functions: MEG helps in understanding which areas of the brain are responsible for specific cognitive functions, like language and memory.
- Epilepsy Diagnosis: MEG can locate epileptic activity, aiding in surgical planning.
- Research: It is used extensively in cognitive neuroscience research to study brain-behavior relationships.
A notable use of MEG is in pre-surgical mapping for epilepsy patients. Surgeons utilize MEG to identify the exact location of the epileptogenic zone, optimizing the success of surgical interventions and minimizing damage to healthy brain tissue.
Advantages of Magnetoencephalography
MEG provides several advantages over other brain imaging techniques, such as:
- High Temporal Resolution: MEG can detect neural activity on a millisecond-by-millisecond basis.
- Non-invasive: Unlike some other imaging techniques, MEG does not require injections or exposure to radiation.
- Direct Measurement: MEG measures magnetic fields directly, offering more accurate timing of neural events than indirect methods.
Unlike Electroencephalography (EEG), which measures electrical activity, MEG measures magnetic fields that are less distorted by the skull and scalp. This means MEG can offer clearer signals from deeper parts of the brain. The combination of both MEG and EEG in some studies helps to cross-validate findings and provide a fuller picture of brain activity.
How Does Magnetoencephalography Work
Magnetoencephalography (MEG) is a highly sophisticated tool that allows scientists to monitor brain activity in real time. This technology offers invaluable insights into the workings of the human brain by detecting the magnetic fields generated by neuronal activity.
The Basics of MEG
Superconducting Quantum Interference Devices (SQUIDs): Extremely sensitive magnetometers used to measure tiny magnetic fields, including those produced by brain activity in MEG.
MEG operates by detecting the magnetic fields produced by electrical currents in your brain. These currents are generated by neural activity and flow through dendrites, the branching extensions of neurons. The small magnetic fields created are detected by SQUID sensors, which are cooled to near absolute zero to maintain their superconductivity.While other brain imaging techniques like EEG measure electrical activity, MEG focuses on the associated magnetic fields, which are less distorted by the skull and scalp.
To understand how MEG can provide high-resolution data, it's essential to delve into the physics of magnetic field detection. The currents in the brain are on the order of femtoamperes. The resulting magnetic fields are in the femtotesla (fT) range, demanding exceptionally sensitive detection methods. This sensitivity allows MEG to achieve temporal resolutions on the order of milliseconds, making it ideal for time-series analysis of brain activity.
Mathematical Concepts in MEG
Mathematics plays a crucial role in MEG, especially in data processing and interpretation. To map brain activity accurately, MEG data undergoes complex mathematical transformations.
For example, the magnetic field produced by a single neuron can be described by Biot-Savart law:\[ \text{B} = \frac{\text{μ}_0}{4π} \frac{\text{I}d\text{L} \times \text{r}}{\text{r}^3} \]where:\[\text{μ}_0\] is the permeability of free space, \[\text{I}\] is the current, \[\text{dL}\] is the length element of the current-carrying wire, and \[\text{r}\] is the position vector from the wire to the point where the field is calculated.
MEG data is often combined with structural MRI data to enhance spatial resolution and provide a comprehensive view of brain activity.
Signal Processing in MEG
Processing MEG signals involves several steps to ensure accurate and meaningful results. Here are the key steps:
- Pre-Processing: Removes artifacts and noise from the raw data.
- Source Localization: Maps the magnetic fields to their corresponding neural sources.
- Data Analysis: Uses statistical and mathematical tools to interpret the activity patterns.
One of the advanced techniques in MEG signal processing is the use of beamforming. Beamforming focuses on reconstructing the neural activity at specific brain locations by optimizing the signal-to-noise ratio. The mathematical foundation of beamforming can be represented by the following equation:\[ \text{Y}(\text{k}) = \text{W}^H (\text{k}) \text{X} (\text{k}) \]where:\[\text{Y} (\text{k})\] is the output signal, \[\text{W}^H (\text{k})\] is the Hermitian transpose of the weight vector, and \[\text{X} (\text{k})\] is the input signal vector.This process results in a more precise localization of brain activity, providing clearer insights into the intricate dynamics of the nervous system.
Magnetoencephalography and Physical Performance
Exploring how magnetoencephalography (MEG) contributes to understanding physical performance can unveil critical insights into the mind-body connection. This section will delve into various aspects of this relationship.
Analyzing Brain Activity During Physical Tasks
MEG allows researchers to study brain activity during physical tasks with high precision. By capturing data in real-time, it reveals how different parts of the brain coordinate movements and respond to physical exertion.Key Points:
- Motor Cortex Activation
- Coordination of Movements
- Reaction Time Analysis
Example: Using MEG, scientists can observe the brain activity of athletes during a simulated sports task. By analyzing the data, they can identify the brain regions responsible for fine motor skills and rapid decision-making.
Impact on Training and Rehabilitation
Understanding the neural basis of physical performance through MEG can play a crucial role in devising more effective training and rehabilitation programs. It can lead to tailored interventions that enhance performance and aid recovery from injuries.Applications:
- Creating Personalized Training Programs
- Improving Rehabilitation Techniques
- Preventing Sports Injuries
MEG data can be used to optimize training schedules by identifying the ideal times for physical exertion and recovery.
Cognitive Load and Physical Performance
Physical tasks often involve significant mental effort, especially in competitive sports. MEG helps in understanding the cognitive load and how it affects physical performance.Observations:
- Effect of Mental Fatigue
- Role of Attention and Focus
- Strategies for Mental Training
Cognitive Strategies: MEG can be employed to study the effects of different cognitive strategies on physical performance. For example, researchers can analyze how visualization techniques improve an athlete’s motor performance. The insights gained can be used to develop mental training programs that enhance both cognitive and physical skills.
Evaluating the Effects of Fatigue
Fatigue, both mental and physical, can significantly impact performance. MEG provides a window into how fatigue affects brain activity, helping to develop methods to mitigate its effects.Key Areas of Research:
- Brain's Response to Fatigue
- Recovery Patterns
- Strategies to Combat Fatigue
Example: In a study on runners, MEG was used to monitor brain activity before and after a long-distance run. The findings showed decreased activity in regions associated with decision-making, indicating how physical exertion impacts cognitive function.
Neuroplasticity and Skill Acquisition
MEG is instrumental in studying neuroplasticity, particularly how the brain adapts and changes during skill acquisition. This is essential for understanding how athletes develop expertise in their chosen sports.Focus Areas:
- Changes in Neural Pathways
- Development of Motor Skills
- Long-term Adaptations
Long-term Changes: By longitudinally tracking athletes using MEG, researchers can observe how continuous practice reorganizes brain networks. This information can help in creating training regimes that maximize brain efficiency and skill retention.
Neuroplasticity insights from MEG can also be applied to non-athletes, aiding in rehabilitation for stroke patients and individuals with motor impairments.
Magnetoencephalography Usage in Sports Science
Magnetoencephalography (MEG) opens new horizons in sports science, providing valuable insights into brain activity and its correlation with physical performance. This section explores how MEG is applied in exercise research and brain electrophysiology.
Magnetoencephalography in Exercise Research
MEG plays a crucial role in exercise research by allowing scientists to observe and analyze brain activity during various physical tasks. This technology helps in understanding the neural mechanisms behind physical performance and endurance.
Example: Consider a study where cyclists' brain activity is monitored using MEG during intense sprints. The findings reveal specific brain regions activated during high-effort phases, enhancing our understanding of mental and physical exertion.
Combining MEG with other imaging techniques like MRI offers more comprehensive data, providing both structural and functional insights.
Deepdive: In studies on endurance athletes, MEG has been used to track changes in brain activity over extended periods of physical exertion. These studies offer insights into how the brain adapts to sustained physical stress, revealing mechanisms of mental resilience.
Magnetoencephalography for Brain Electrophysiology and Imaging
MEG also provides high-resolution data on brain electrophysiology and imaging. This capability is essential for mapping brain functions and understanding the intricate details of neural activity.
Electrophysiology: The study of electrical properties and activities within biological cells and tissues, especially neurons.
Example: By using MEG, researchers can study the electrophysiological responses of the brain during complex motor tasks, helping in the development of interventions to improve athletic performance.
Deepdive: Advanced MEG techniques, such as time-frequency analysis, allow for detailed examination of brain rhythms and their role in motor control. These analyses help in understanding how different frequencies correlate with specific cognitive and physical tasks.
MEG's ability to capture real-time data makes it an invaluable tool for studying rapid neural processes.
To sum up, MEG has proven to be a versatile tool in the fields of exercise research and brain electrophysiology. Its high temporal resolution and non-invasive nature make it ideal for studying the dynamic aspects of brain activity.
Magnetoencephalography Advantages and Disadvantages
Magnetoencephalography (MEG) offers a wealth of benefits and some drawbacks in its application in neuroscience and medical fields. Understanding both sides can help you make informed decisions on its use.
Advantages of Magnetoencephalography
MEG stands out due to several key advantages, including its high temporal resolution and non-invasive nature.
- High Temporal Resolution: MEG can detect neural activity on a millisecond-by-millisecond basis, enabling precise tracking of brain functions.
- Non-invasive: Unlike other imaging techniques, MEG does not require injections or exposure to radiation, making it safer for repeated use.
- Direct Measurement: MEG measures magnetic fields directly, offering more accurate timing of neural events compared to indirect methods.
- High Sensitivity: The sensitivity of MEG allows for the detection of tiny magnetic fields produced by neuronal activity.
Example: Consider a study where MEG is used to monitor brain activity during language processing. The high temporal resolution allows researchers to pinpoint the exact time window when specific brain regions are active, providing detailed insights into linguistic processing.
Deepdive: One of the advanced applications of MEG is in detecting and analyzing brain oscillations. By using time-frequency analysis, researchers can observe the interaction between different brain regions in real-time. This technique helps in understanding complex neural dynamics, such as those involved in decision-making and emotional regulation.
Disadvantages of Magnetoencephalography
While MEG offers numerous benefits, it also has some limitations that you should be aware of.
- High Cost: MEG systems are extremely expensive to purchase and maintain, which can limit their accessibility.
- Magnetic Shielding: The requirement for a magnetically shielded room to block external interference can be a logistical challenge.
- Spatial Resolution: MEG provides excellent temporal resolution but has comparatively lower spatial resolution compared to fMRI.
- Complex Data Analysis: The data produced by MEG is complex and requires specialized knowledge and software for analysis.
Combining MEG with fMRI can help overcome its spatial resolution limitation, providing a comprehensive view of both timing and location of brain activity.
Example: In clinical settings, MEG is often used for pre-surgical mapping of brain tumors. The high temporal resolution helps in identifying critical functional areas, although the need for additional spatial imaging such as MRI is usually necessary to get a complete picture.
Deepdive: Despite its challenges, MEG remains invaluable in specific research areas like epilepsy. In these cases, its ability to accurately localize epileptic foci in the brain makes it essential for planning surgical interventions. Detailed mathematical models and algorithms are employed to interpret the intricate data from MEG, which can guide life-saving operations.
Magnetoencephalography - Key takeaways
- Magnetoencephalography (MEG): A brain imaging technique that measures the magnetic fields generated by neuronal activity, providing high temporal resolution.
- How MEG Works: Utilizes Superconducting Quantum Interference Devices (SQUIDs) to detect magnetic fields produced by electrical activity in the brain.
- Applications: Used for mapping brain functions, epilepsy diagnosis, and cognitive neuroscience research.
- Advantages and Disadvantages: High temporal resolution and non-invasive, but expensive and requires magnetic shielding.
- Usage in Sports Science: Offers insights into brain activity during physical tasks, aiding in training, rehabilitation, and understanding fatigue and neuroplasticity.
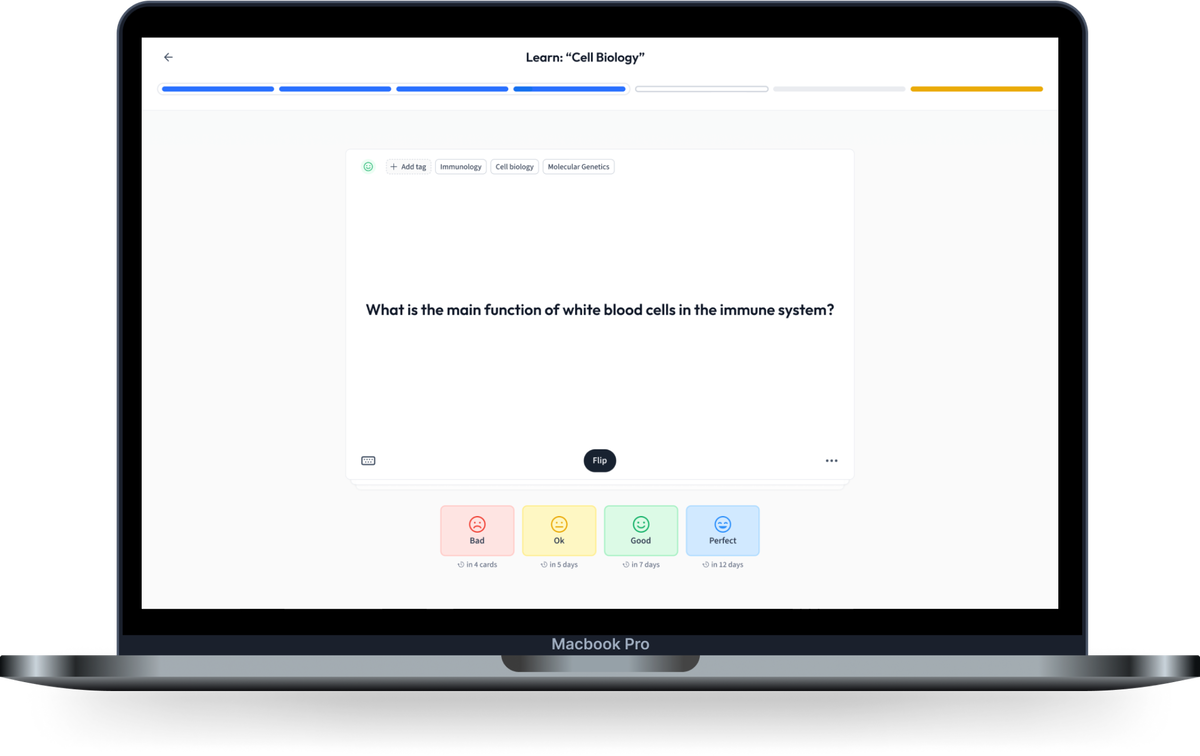
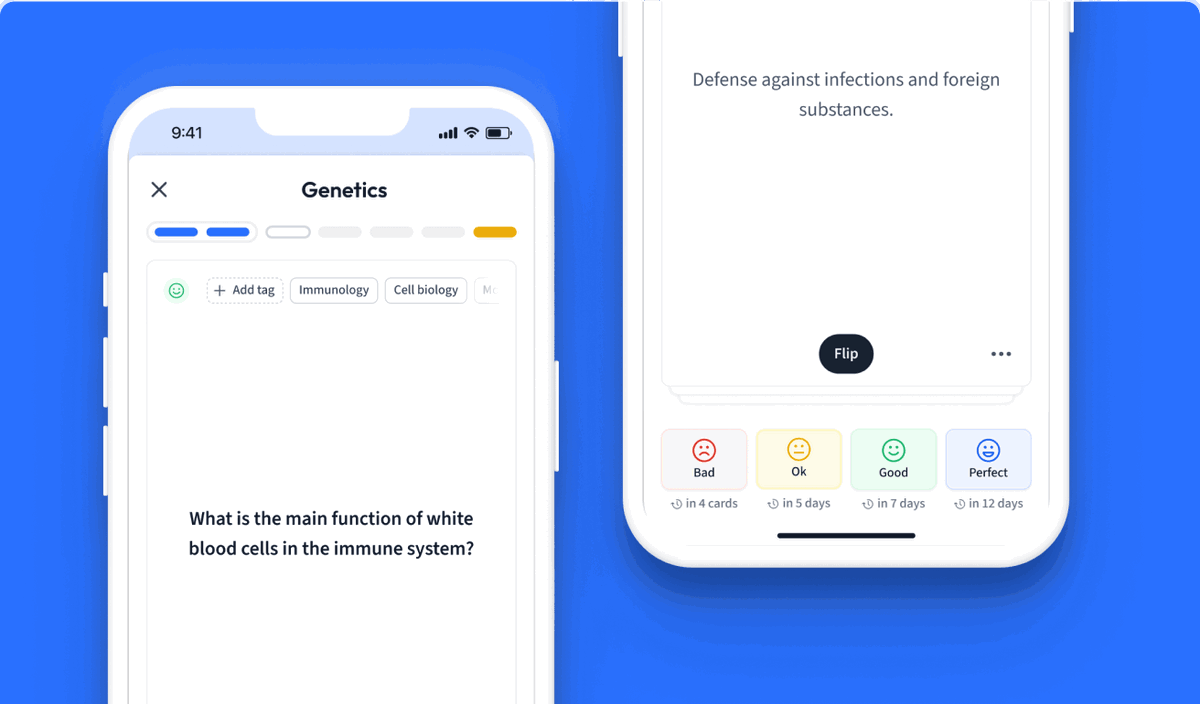
Learn with 15 Magnetoencephalography flashcards in the free StudySmarter app
Already have an account? Log in
Frequently Asked Questions about Magnetoencephalography
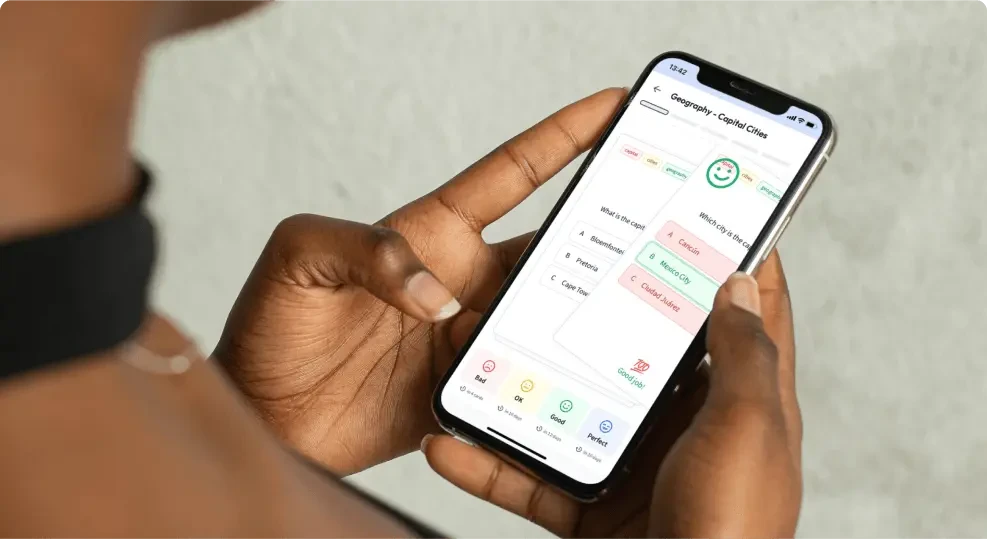
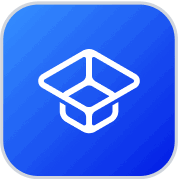
About StudySmarter
StudySmarter is a globally recognized educational technology company, offering a holistic learning platform designed for students of all ages and educational levels. Our platform provides learning support for a wide range of subjects, including STEM, Social Sciences, and Languages and also helps students to successfully master various tests and exams worldwide, such as GCSE, A Level, SAT, ACT, Abitur, and more. We offer an extensive library of learning materials, including interactive flashcards, comprehensive textbook solutions, and detailed explanations. The cutting-edge technology and tools we provide help students create their own learning materials. StudySmarter’s content is not only expert-verified but also regularly updated to ensure accuracy and relevance.
Learn more