Jump to a key chapter
Definition of Biomechanics Modeling
Biomechanics Modeling is a concept used in sports science to analyze the mechanical aspects of human movement. This field applies principles from mechanics, such as motion and forces, to biological systems, particularly focusing on how muscles, bones, tendons, and ligaments work together to produce movement.
Importance of Biomechanics Modeling in Sports Science
Understanding Biomechanics Modeling is crucial in sports science due to its various applications. By studying the mechanics of human movement, you can:
- Improve athletic performance.
- Prevent injuries by identifying hazardous movement patterns.
- Develop effective training programs.
- Rehabilitate sports-related injuries.
Key Concepts in Biomechanics Modeling
To get started with Biomechanics Modeling, it is essential to grasp some key concepts:
- Kinematics: The study of motion without considering the forces that cause it. Key variables include position, velocity, and acceleration.
- Kinetics: The study of the forces that cause motion, including muscle forces, gravity, and friction.
- Statistical Modeling: Utilizing data to build models that predict or describe human movements.
- Computational Modeling: Using computer simulations to analyze and visualize movements.
Biomechanics Modeling: The application of mechanical principles to biological systems for the analysis of movement and forces in living organisms.
Mathematical Equations in Biomechanics
Mathematical equations are fundamental to Biomechanics Modeling. They help describe and quantify the motion and forces in the body. Key equations include:
- Newton's Second Law of Motion: \(F = ma\) where \(F\) is the force applied, \(m\) is mass, and \(a\) is acceleration.
- Equation of Motion: Utilized to describe the motion of a body given forces and moments. E.g., \(\frac{d}{dt}(mv)=F\).
- Wolff's Law: Describes how bone density changes in response to mechanical stress. Often simplified as \(O = W \frac{dm} {dx}\), where \(W\) is the applied load, and \(\frac{dm}{dx}\) is the rate of bone density change.
Consider how runners benefit from Biomechanics Modeling. By analyzing a runner's form and technique using computational models, you can pinpoint inefficiencies. For instance, if the model shows excessive vertical motion, it can be concluded that the runner is wasting energy moving up and down rather than forward. Thus, adjustments can be made to the running technique to improve efficiency.
For students interested in the mechanics of the musculoskeletal system, a deep dive into inverse dynamics could be enlightening. Inverse dynamics involves calculating the forces and moments needed to produce observed movements. This approach is commonly used in gait analysis, where movements are recorded, and the required joint torques are computed. Using this technique can uncover subtle imbalances or misalignments that may not be obvious otherwise.
Hint: Always validate your models with experimental data to ensure accuracy and reliability.
Biomechanics Modeling in Sports Science
Biomechanics Modeling is a concept used in sports science to analyze the mechanical aspects of human movement. This field applies principles from mechanics, such as motion and forces, to biological systems, particularly focusing on how muscles, bones, tendons, and ligaments work together to produce movement.
Importance of Biomechanics Modeling in Sports Science
Understanding Biomechanics Modeling is crucial in sports science due to its various applications. By studying the mechanics of human movement, you can:
- Improve athletic performance.
- Prevent injuries by identifying hazardous movement patterns.
- Develop effective training programs.
- Rehabilitate sports-related injuries.
Key Concepts in Biomechanics Modeling
To get started with Biomechanics Modeling, it is essential to grasp some key concepts:
- Kinematics: The study of motion without considering the forces that cause it. Key variables include position, velocity, and acceleration.
- Kinetics: The study of the forces that cause motion, including muscle forces, gravity, and friction.
- Statistical Modeling: Utilizing data to build models that predict or describe human movements.
- Computational Modeling: Using computer simulations to analyze and visualize movements.
Biomechanics Modeling: The application of mechanical principles to biological systems for the analysis of movement and forces in living organisms.
Mathematical Equations in Biomechanics
Mathematical equations are fundamental to Biomechanics Modeling. They help describe and quantify the motion and forces in the body. Key equations include:
- Newton's Second Law of Motion: \(F = ma\) where \(F\) is the force applied, \(m\) is mass, and \(a\) is acceleration.
- Equation of Motion: Utilized to describe the motion of a body given forces and moments. E.g., \( \frac{d}{dt}(mv)= F \).
- Wolff's Law: Describes how bone density changes in response to mechanical stress. Often simplified as \( O = W \frac{dm}{dx} \), where \( W \) is the applied load, and \( \frac{dm}{dx} \) is the rate of bone density change.
Consider how runners benefit from Biomechanics Modeling. By analyzing a runner's form and technique using computational models, you can pinpoint inefficiencies. For instance, if the model shows excessive vertical motion, it can be concluded that the runner is wasting energy moving up and down rather than forward. Thus, adjustments can be made to the running technique to improve efficiency.
For students interested in the mechanics of the musculoskeletal system, a deep dive into inverse dynamics could be enlightening. Inverse dynamics involves calculating the forces and moments needed to produce observed movements. This approach is commonly used in gait analysis, where movements are recorded, and the required joint torques are computed. Using this technique can uncover subtle imbalances or misalignments that may not be obvious otherwise.
Hint: Always validate your models with experimental data to ensure accuracy and reliability.
Biomechanics Modeling Techniques
Biomechanics Modeling Techniques focus on various methods to analyze human movement and the mechanical processes involved. These techniques are vital in understanding how to improve athletic performance and prevent injuries.
Computational Modeling in Biomechanics
Computational modeling uses computer simulations to replicate and study the biomechanics of human movement. The primary advantage of computational modeling is its ability to handle complex data and perform detailed analysis quickly.
Computational Modeling: The use of computer algorithms and simulations to analyze and predict the mechanics of biological systems.
In computational modeling, several mathematical equations are used:
- Newton's Laws of Motion: These laws describe the relationship between the motion of an object and the forces acting on it. For instance, \(F = ma\), where \(F\) is the force applied, \(m\) is mass, and \(a\) is acceleration.
- Inverse Dynamics: This method involves calculating the forces and moments required to produce observed movements. It often uses equations like \( \tau = I \dot{\omega} + \omega \times (I \omega)\), where \( \tau\) is torque, \(I \) is inertia, and \( \omega\) is angular velocity.
Consider a football player kicking a ball. By applying inverse dynamics, you can calculate the torque required at the knee joint to achieve the kicking motion. This helps in understanding the stress on the knee and in designing training programs to strengthen the joint.
For those interested in computational modeling, exploring Finite Element Analysis (FEA) is worthwhile. FEA divides a complex structure into smaller parts called elements. Each element's behavior is predicted using equations, and then these predictions are combined to understand the overall behavior. This technique is particularly useful in studying stress distribution within bones or muscles under different loading conditions.
Data Collection Methods
Accurate data collection is critical in Biomechanics Modeling to ensure reliable results. Various methods are employed to gather data on human movement and the forces involved.
Data Collection: The process of gathering, measuring, and analyzing accurate data to support biomechanics modeling and analysis.
Common data collection methods include:
- Motion Capture Systems: Use cameras and markers placed on the body to track movements in three-dimensional space. These systems provide precise data on joint angles, velocities, and accelerations.
- Force Plates: Measure the ground reaction forces experienced during movements such as walking or jumping. These data are vital for understanding the kinetics of motion.
- Electromyography (EMG): Records the electrical activity produced by muscles. This method helps in studying muscle activation patterns during various activities.
Hint: Combining multiple data collection methods can provide a more comprehensive understanding of biomechanical processes.
Diving deeper, you can explore wearable technology such as inertial measurement units (IMUs). These devices use accelerometers, gyroscopes, and magnetometers to provide detailed data on body movement. Wearable tech is advantageous as it allows for data collection in real-world settings outside a lab environment.
Simulation and Analysis
Simulation and analysis are the final steps in Biomechanics Modeling. Once data is collected and models are created, simulations can be run to predict outcomes and analyze various scenarios.
Simulation: The process of using a model to run experiments and predict the behavior of a biomechanical system under different conditions.
Simulations often involve running virtual experiments to test hypotheses. This can include:
- Predicting injury risks by simulating high-stress activities and identifying weak points.
- Optimizing performance by testing different techniques or equipment in a virtual environment.
- Developing rehabilitation programs by simulating the effects of various treatments on injury recovery.
Consider simulating the effect of different shoe designs on a runner's biomechanics. By altering the shoe's properties in the simulation, you can determine which design offers the best performance and injury prevention benefits.
For those interested, delving into musculoskeletal modeling can provide further insights. Musculoskeletal models simulate the internal mechanics of the body, such as muscle forces and joint reactions. These models are used extensively in gait analysis, sports performance studies, and ergonomic assessments.
Applications of Biomechanics Modeling
Biomechanics Modeling has diverse applications in the sports science field. These applications help athletes improve their performance, prevent injuries, and rehabilitate effectively.
Performance Enhancement
Biomechanics Modeling can significantly enhance athletic performance. By analyzing movement patterns, muscle activity, and joint mechanics, you can identify areas of improvement and develop strategies for optimizing performance.
Performance Enhancement: The process of improving athletic abilities through various methods, including biomechanical analysis.
Some key techniques for enhancing performance include:
- Motion Analysis: Tracking and analyzing the movement of athletes to enhance techniques.
- Force Measurement: Using force plates to measure ground reaction forces and improve efficiency in movements.
- Equipment Optimization: Customizing sports equipment based on biomechanical data.
Consider a swimmer looking to reduce drag and improve stroke efficiency. By using motion capture technology, you can analyze the swimmer's stroke mechanics and recommend adjustments to arm angles or kick timing for better performance.
Hint: Always use a combination of motion analysis and force measurement to get a comprehensive understanding of the athlete's performance.
Injury Prevention
Biomechanics Modeling plays a crucial role in preventing sports injuries by identifying hazardous movement patterns and mechanical imbalances.
Injury Prevention: The practice of identifying and mitigating risk factors to avoid sports-related injuries.
Key techniques for injury prevention include:
- Gait Analysis: Examining walking and running patterns to identify misalignments that could lead to injuries.
- Stress Analysis: Using computational models to understand the impact of different stresses on the body.
- Strength and Flexibility Testing: Assessing muscle strength and joint flexibility to identify weaknesses.
A basketball player frequently experiencing knee pain might use gait analysis to reveal that they have an inefficient landing technique. By adjusting their landing mechanics, the risk of knee injuries can be reduced.
Diving deeper into stress analysis, you can use Finite Element Analysis (FEA) to study how different loads affect bones and tissues. This can help in designing better training regimens and equipment that minimizes injury risks.
Hint: Regular assessments can help in early detection of potential injury risks, allowing for timely intervention.
Rehabilitation
In the context of sports science, Biomechanics Modeling is instrumental in designing effective rehabilitation programs for injured athletes.
Rehabilitation: The process of restoring an athlete's normal function and performance following an injury.
Key techniques in rehabilitation include:
- Range of Motion Measurement: Assessing the flexibility of joints to track recovery progress.
- Muscle Strength Testing: Evaluating the strength of affected muscles to guide rehabilitation exercises.
- Biomechanical Feedback: Providing real-time feedback on movement patterns to ensure proper technique during recovery exercises.
For a soccer player recovering from an ankle injury, range of motion measurement and muscle strength testing can help tailor a rehabilitation program that gradually reintroduces sports-specific drills while minimizing the risk of re-injury.
A deeper exploration into biomechanical feedback can involve the use of wearable sensors that provide real-time data on an athlete's movements. By continuously monitoring this data, you can make on-the-fly adjustments to the rehabilitation regimen, ensuring optimal recovery.
Biomechanics Modeling Explained
Biomechanics Modeling is a powerful tool in sports science that analyzes the mechanical aspects of human movement. By applying principles from mechanics, such as motion and forces, to biological systems, you can gain insights into how muscles, bones, tendons, and ligaments work together to produce movement.
Importance of Biomechanics Modeling
Understanding Biomechanics Modeling offers several benefits in sports science. You can use it to:
- Improve athletic performance.
- Prevent injuries by identifying hazardous movement patterns.
- Develop effective training programs.
- Rehabilitate sports-related injuries.
Key Concepts in Biomechanics Modeling
Biomechanics Modeling involves several key concepts that you should understand:
- Kinematics: The study of motion without considering the forces that cause it. This includes variables such as position, velocity, and acceleration.
- Kinetics: The study of the forces that cause motion. This includes muscle forces, gravitational forces, and frictional forces.
- Statistical Modeling: Utilizing data to build models that predict or describe human movements.
- Computational Modeling: Using computer simulations to analyze and visualize movements.
Biomechanics Modeling: The application of mechanical principles to biological systems for the analysis of movement and forces in living organisms.
Mathematical Foundations in Biomechanics
Mathematics is crucial in Biomechanics Modeling. You use mathematical equations to describe and quantify the motion and forces in the body. Here are some key equations:
- Newton's Second Law of Motion: \(F = ma\) where \(F\) is the force applied, \(m\) is mass, and \(a\) is acceleration.
- Equation of Motion: Utilized to describe the motion of a body given forces and moments. For instance, \( \frac{d}{dt}(mv)= F \).
- Wolff's Law: Describes how bone density changes in response to mechanical stress. Often simplified as \( O = W \frac{dm}{dx} \), where \( W \) is the applied load, and \( \frac{dm}{dx} \) is the rate of bone density change.
Consider an example of how runners benefit from Biomechanics Modeling. By analyzing a runner's form and technique using computational models, you can pinpoint inefficiencies. For instance, if the model shows excessive vertical motion, it indicates that the runner wastes energy moving up and down rather than forward. By making adjustments to the running technique, the efficiency can be improved.
For those interested in the mechanics of the musculoskeletal system, a deep dive into inverse dynamics could be enlightening. Inverse dynamics involves calculating the forces and moments needed to produce observed movements. This approach is commonly used in gait analysis, where movements are recorded, and the required joint torques are computed. Using this technique can uncover subtle imbalances or misalignments that may not be obvious otherwise.
Hint: Always validate your models with experimental data to ensure accuracy and reliability.
Biomechanics Modeling - Key takeaways
- Definition of Biomechanics Modeling: Application of mechanical principles to biological systems for analyzing movement and forces in living organisms.
- Importance of Biomechanics Modeling in Sports Science: Enhances athletic performance, prevents injuries, develops training programs, and aids in rehabilitation.
- Key Concepts: Includes kinematics (study of motion without forces), kinetics (study of forces that cause motion), statistical modeling, and computational modeling (using computer simulations).
- Mathematical Equations: Important equations like Newton's Second Law (\textit{F} = \textit{ma}), Equation of Motion (\textit{d}/\textit{dt}(\textit{mv}) = \textit{F}), and Wolff's Law (bone density changes under stress).
- Applications of Biomechanics Modeling: Analyze running techniques, perform gait analysis, and study rehabilitation processes through tools like inverse dynamics and computational simulations.
Learn faster with the 15 flashcards about Biomechanics Modeling
Sign up for free to gain access to all our flashcards.
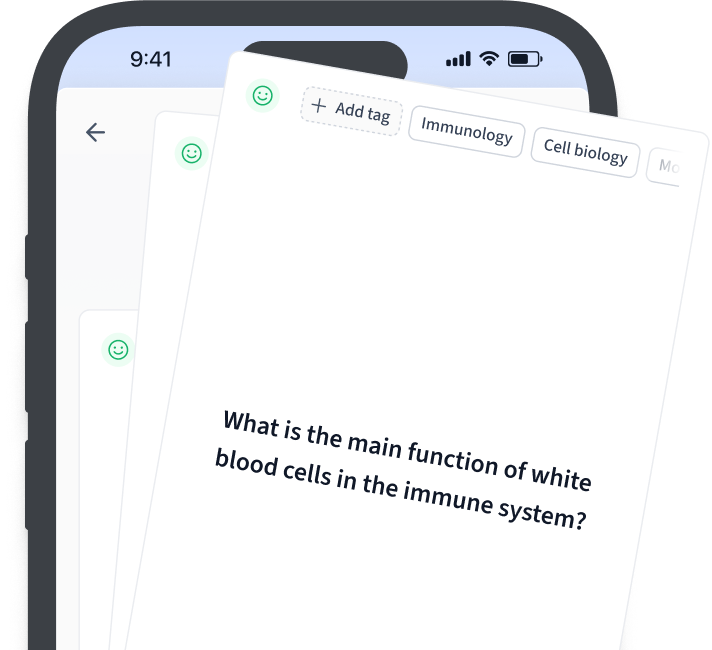
Frequently Asked Questions about Biomechanics Modeling
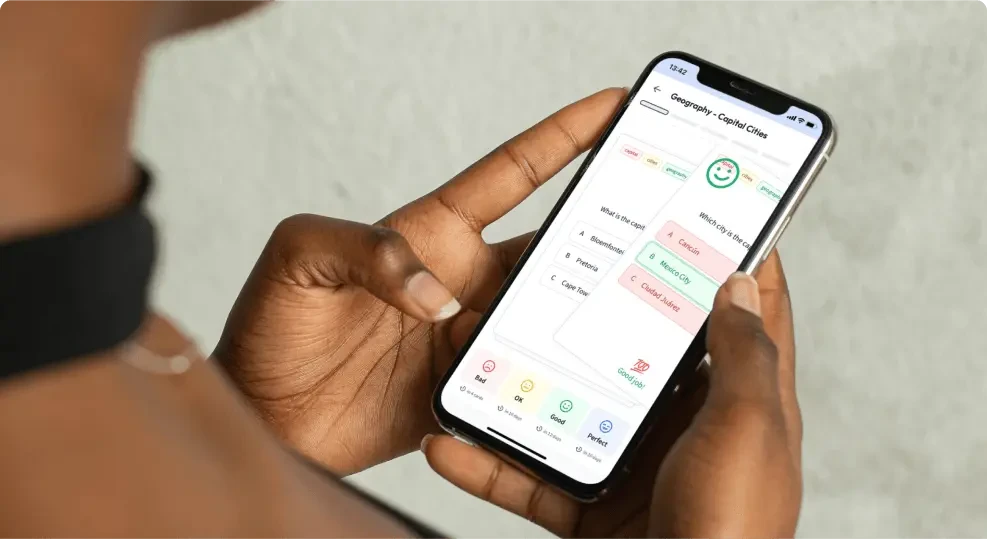
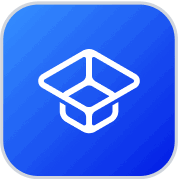
About StudySmarter
StudySmarter is a globally recognized educational technology company, offering a holistic learning platform designed for students of all ages and educational levels. Our platform provides learning support for a wide range of subjects, including STEM, Social Sciences, and Languages and also helps students to successfully master various tests and exams worldwide, such as GCSE, A Level, SAT, ACT, Abitur, and more. We offer an extensive library of learning materials, including interactive flashcards, comprehensive textbook solutions, and detailed explanations. The cutting-edge technology and tools we provide help students create their own learning materials. StudySmarter’s content is not only expert-verified but also regularly updated to ensure accuracy and relevance.
Learn more