Jump to a key chapter
Muscle Biochemistry Overview
Understanding muscle biochemistry is key to grasping how muscles function and respond to various stimuli. This knowledge is particularly beneficial for athletes, medical professionals, and anyone interested in improving physical fitness.
Basic Concepts in Muscle Biochemistry
Muscle biochemistry involves the study of the chemical processes and substances that are crucial for muscle function. It primarily focuses on the molecules that provide the energy required for muscle contractions and growth. At the cellular level, muscles are made up of long fibers that contain many smaller units called myofibrils. These myofibrils consist of repeating sections called sarcomeres, which are the basic functional units of muscle fibers.
Proteins like actin and myosin play critical roles in muscle contraction.
Several key molecules are involved in muscle biochemistry:
- Adenosine triphosphate (ATP): The main energy currency of the cell.
- Creatine phosphate: A molecule that helps quickly regenerate ATP in muscles.
- Glycogen and Glucose: Important sources of energy derived from carbohydrates.
- Fatty acids and Triglycerides: Energy sources from fats.
When exploring muscle biochemistry, it is important to understand the role of enzymes. Enzymes are proteins that act as catalysts to speed up chemical reactions. In muscle cells, key enzymes like Creatine kinase and Lactate dehydrogenase are essential for energy production. Creatine kinase facilitates the conversion between creatine phosphate and ATP, while Lactate dehydrogenase is involved in converting pyruvate to lactate during anaerobic respiration.
ATP Role in Muscle Biochemistry
ATP, or Adenosine triphosphate, is often referred to as the energy currency of the cell because it stores and transfers energy needed for various cellular processes. In muscle biochemistry, ATP is essential for muscle contraction and relaxation.
ATP: A high-energy molecule found in every cell. Its job is to store and supply the cell with needed energy.
When you lift a weight, ATP is used to detach the myosin heads from the actin filaments in your muscle fibers, allowing another cycle of muscle contraction.
The body stores limited amounts of ATP, so it must constantly be regenerated. There are three primary systems for ATP regeneration:
- Phosphagen System: Uses creatine phosphate to quickly regenerate ATP.
- Glycolytic System: Uses glucose or glycogen to produce ATP through glycolysis, resulting in pyruvate or lactate.
- Oxidative System: Uses oxygen to produce ATP through the processes of the Krebs cycle and the electron transport chain.
The phosphagen system is the fastest way to regenerate ATP, but it only lasts for about 10 seconds.
ATP hydrolysis is the process of breaking down ATP into adenosine diphosphate (ADP) and an inorganic phosphate molecule. This breakdown releases energy, which is then used for muscle contractions. To maintain ATP levels, ADP can be reconverted to ATP through phosphorylation, which can be facilitated via substrate-level phosphorylation, oxidative phosphorylation, or photophosphorylation (in plants). In muscles, substrate-level phosphorylation occurs in the cytosol during glycolysis and is a direct method of regenerating ATP. Oxidative phosphorylation happens in the mitochondria and involves a series of reactions in the electron transport chain. Together, these processes ensure a continuous supply of ATP to fuel muscular activities and are particularly important in extended or intense exercises.
Biochemistry of Muscle Contraction
Muscle contraction is a complex process involving numerous biochemical reactions. Understanding these mechanisms will provide you with better insights into how muscles work during physical activities.
Mechanisms of Muscle Contraction
Muscle contraction occurs through a series of well-coordinated events. The central concept behind muscle contraction is the sliding filament theory, which involves the sliding of actin and myosin filaments past each other to produce a contraction.
The sliding filament theory explains how thick (myosin) and thin (actin) filaments slide past one another during muscle contraction.
Here are the key steps involved in muscle contraction:
- Nerve impulses trigger the release of calcium ions (Ca2+) from the sarcoplasmic reticulum.
- Calcium ions bind to troponin, causing a change in its shape.
- This shape change moves tropomyosin, uncovering binding sites on actin filaments.
- Myosin heads, powered by ATP hydrolysis, attach to these binding sites, forming cross-bridges.
- The power stroke occurs as the myosin heads pivot, pulling actin filaments toward the center of the sarcomere.
- ATP binds to myosin, causing it to detach from actin and be ready for another cycle.
One fascinating aspect of muscle contraction is the role of Calcium ions (Ca2+). These ions act as a regulatory signal that initiates the contraction process. When a nerve impulse reaches the neuromuscular junction, it triggers the influx of calcium ions into the muscle cell. These ions bind to troponin, causing a conformational change that moves tropomyosin away from actin's binding sites. This allows myosin heads to interact with actin, leading to muscle contraction. Calcium ions are then quickly pumped back into the sarcoplasmic reticulum, allowing the muscle to relax. This rapid cycling of calcium is crucial for the precision and timing of muscle contractions.
Molecular Players in Muscle Contraction
Several molecules and proteins play critical roles in muscle contraction. The main molecular players include actin, myosin, troponin, and tropomyosin. Let's explore the roles of these molecules in detail.
Actin: A protein that forms thin filaments and interacts with myosin during muscle contraction.
Myosin: A protein that forms thick filaments and interacts with actin to generate muscle contraction forces.
When an impulse triggers muscle contraction, myosin heads bind to actin filaments to form a cross-bridge. Utilizing the energy from ATP, myosin heads pull actin filaments toward the center of the sarcomere, resulting in muscle shortening.
Here's a closer look at the molecular players:
- Actin: Forms the thin filaments and provides a site for myosin to attach.
- Myosin: Comprises thick filaments with heads that bind to actin to perform the power stroke.
- Troponin: A regulatory protein that binds to calcium ions and causes the movement of tropomyosin.
- Tropomyosin: A protein that blocks myosin binding sites on actin in relaxed muscles.
Another important player in muscle biochemistry is ATPase enzymes in myosin heads. These enzymes hydrolyze ATP to ADP and inorganic phosphate, releasing energy needed for muscle contraction. The rate at which ATPase operates can affect the speed and strength of muscle contractions. Fast-twitch muscle fibers contain myosin ATPase that works rapidly, providing quick and powerful contractions suitable for sprinting. In contrast, slow-twitch muscle fibers have a slower ATPase, which provides endurance and sustained contractions required for activities like long-distance running.
Muscle Metabolism Biochemistry
Muscle metabolism biochemistry is essential for understanding how your muscles generate energy and perform work. This section focuses on the biochemical pathways that enable muscles to produce and utilize energy efficiently.
Energy Pathways in Muscle Cells
Your muscle cells have various energy pathways to generate ATP, the primary energy carrier. These pathways allow for immediate, short-term, and long-term energy production to meet different activity levels and durations.
Adenosine triphosphate (ATP): The primary energy carrier in cells that stores and transfers energy for various biochemical reactions.
Muscle cells use three main energy pathways:
- Phosphagen System: Uses creatine phosphate to quickly regenerate ATP, ideal for high-intensity, short-duration activities.
- Glycolytic System: Breaks down glucose or glycogen to produce ATP through glycolysis, suitable for moderate-intensity activities lasting up to a few minutes.
- Oxidative System: Uses oxygen to produce ATP via the Krebs cycle and electron transport chain, supporting long-duration, low-intensity activities.
When sprinting, your muscles primarily rely on the phosphagen system for quick ATP regeneration. For a 400-meter run, the glycolytic system kicks in, while marathon runners depend on the oxidative system for sustained ATP production.
The oxidative phosphorylation in the mitochondria is a complex yet efficient process for producing ATP. This process involves a series of electron carriers and enzymes known as the electron transport chain. Electrons travel down this chain, releasing energy that pumps protons across the mitochondrial membrane to create a proton gradient. The energy from this gradient drives the synthesis of ATP from ADP and inorganic phosphate through an enzyme called ATP synthase. The oxidative system's efficiency makes it vital for endurance activities and continuous energy supply for muscle functions.
The phosphagen system can replenish ATP the fastest but is exhausted quickly, usually within 10 seconds.
ATP Production and Utilization in Muscles
Muscles require a continuous supply of ATP to function efficiently. Understanding how ATP is produced and utilized helps you better appreciate the complexity of muscle biochemistry.
ATP production in muscles primarily involves three pathways:
- Phosphagen System: Rapid ATP generation using creatine phosphate.
- Glycolytic System: Moderate ATP production from glycolysis of glucose or glycogen.
- Oxidative System: High-yield ATP production via oxidative phosphorylation in mitochondria.
During a weightlifting session, ATP is generated quickly through the phosphagen system, allowing your muscles to contract forcefully. In contrast, a long-distance run relies on the oxidative system to provide a sustained ATP supply for prolonged muscle activity.
In the glycolytic system, glucose is broken down into pyruvate through a series of enzymatic reactions. In anaerobic conditions, pyruvate is converted into lactate, allowing glycolysis to continue and generate ATP without oxygen. However, this leads to a buildup of lactate, which can cause muscle fatigue. In aerobic conditions, pyruvate enters the mitochondria and is used in the Krebs cycle to produce more ATP through oxidative phosphorylation. This efficient ATP generation highlights the versatility and adaptability of muscle metabolism based on oxygen availability and activity intensity.
The oxidative system is also known as aerobic metabolism, as it relies on oxygen to produce ATP.
Biochemistry of Muscle Fatigue
Understanding the biochemistry of muscle fatigue is crucial for recognizing why muscles become tired and how this impacts performance. Muscle fatigue involves several biochemical changes within your muscle cells.
Causes of Muscle Fatigue
Muscle fatigue arises from multiple factors that alter biochemical processes within muscle fibers. Some of the common causes include:
- Accumulation of Metabolites: Exercise induces the accumulation of metabolites such as ADP, inorganic phosphate (Pi), and hydrogen ions (H+), which can interfere with muscle contractions.
- Depletion of Energy Stores: Prolonged exercise depletes ATP and glycogen stores, limiting the muscle's ability to sustain contractions.
- Impaired Calcium Release: Reduced release of calcium ions from the sarcoplasmic reticulum can diminish muscle contraction efficiency.
When you engage in high-intensity exercise, the buildup of lactic acid and hydrogen ions lowers the pH in your muscle cells, leading to a sensation of burning and a decline in muscle performance.
Adequate hydration and proper nutrient intake can help delay muscle fatigue.
Exploring the role of the enzyme Na+/K+-ATPase reveals its significance in muscle fatigue. This enzyme maintains the electrochemical gradients of sodium and potassium ions across the muscle cell membrane, essential for action potential propagation and muscle excitation. During prolonged exercise, the function of Na+/K+-ATPase can become compromised, leading to ion imbalances. Such imbalances result in reduced muscle excitability and contribute to fatigue. Moreover, prolonged activity might also compromise membrane potential maintenance, making it harder for muscles to contract efficiently.
Impact of Muscle Fatigue on Performance
Muscle fatigue significantly impacts physical performance and can limit athletic activities:
- Reduced Strength: Fatigued muscles generate less force and power, affecting the ability to perform high-intensity tasks.
- Decreased Endurance: The onset of muscle fatigue lowers stamina, making it difficult to sustain prolonged physical efforts.
- Coordination and Accuracy: Fatigue can impair motor unit coordination, leading to decreased precision and potentially increasing the risk of injury.
During a marathon, muscle fatigue can cause a runner to slow down in the later stages of the race, reducing overall performance and finish time.
Regular training and proper recovery strategies can enhance muscle endurance and delay the onset of fatigue.
At a molecular level, muscle fatigue is closely linked to oxidative stress. Reactive oxygen species (ROS) are byproducts of cellular respiration that can damage cellular components when not adequately controlled. During intense or prolonged exercise, the production of ROS increases, overwhelming the antioxidant defenses and leading to oxidative stress. This stress can damage proteins, lipids, and DNA within muscle cells, exacerbating the decline in muscle function and contributing to fatigue. Antioxidants such as vitamins C and E, along with compounds found in certain foods, can help mitigate oxidative stress and improve muscle performance.
Muscle Biochemistry - Key takeaways
- Muscle Biochemistry: The study of chemical processes and substances crucial for muscle function, energy, and growth.
- ATP Role in Muscle Biochemistry: ATP is essential for muscle contraction and relaxation, acting as the cell's main energy currency.
- Biochemistry of Muscle Contraction: Involves actin and myosin interactions, regulated by calcium ions, troponin, and tropomyosin.
- Muscle Metabolism Biochemistry: Involves pathways like the Phosphagen, Glycolytic, and Oxidative systems for ATP generation based on activity intensity and duration.
- Biochemistry of Muscle Fatigue: Caused by metabolite accumulation, energy store depletion, and impaired calcium release, impacting muscle performance and endurance.
Learn faster with the 12 flashcards about Muscle Biochemistry
Sign up for free to gain access to all our flashcards.
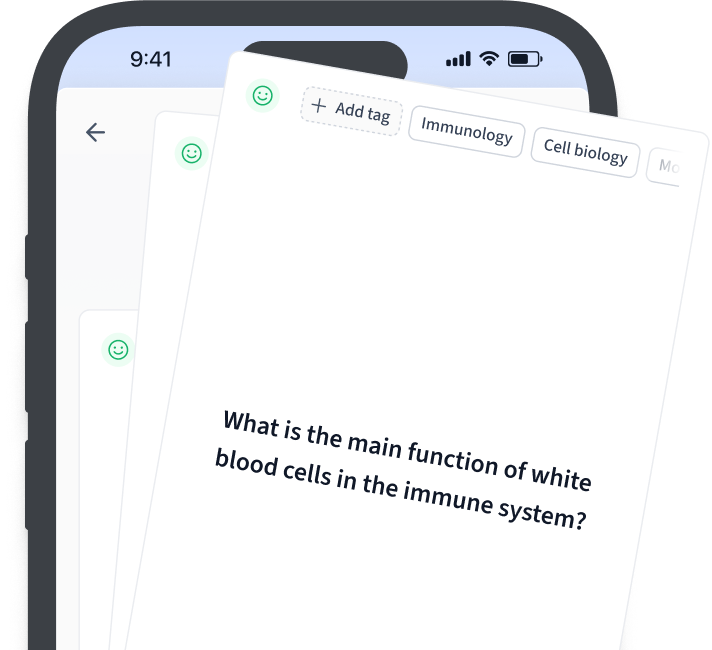
Frequently Asked Questions about Muscle Biochemistry
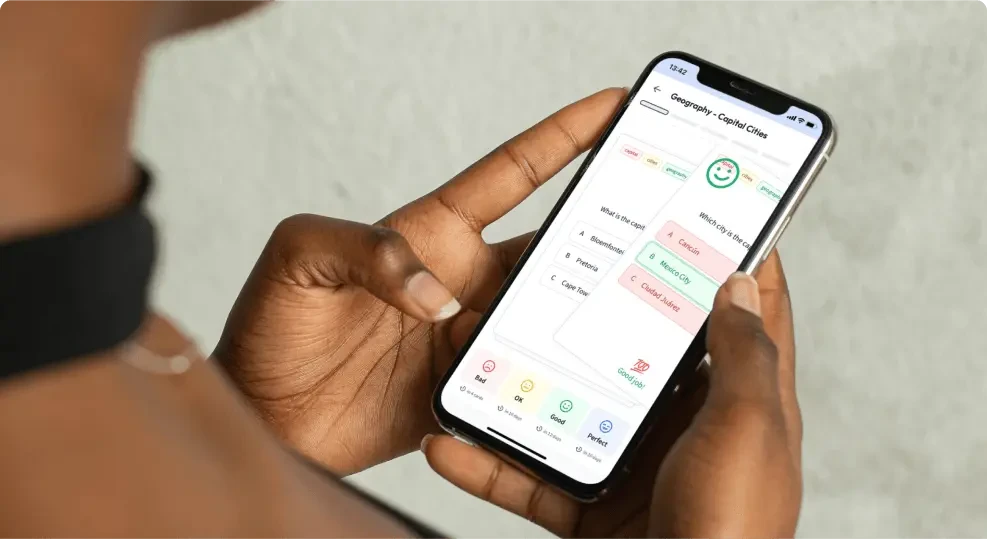
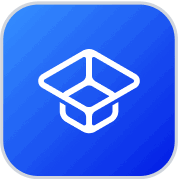
About StudySmarter
StudySmarter is a globally recognized educational technology company, offering a holistic learning platform designed for students of all ages and educational levels. Our platform provides learning support for a wide range of subjects, including STEM, Social Sciences, and Languages and also helps students to successfully master various tests and exams worldwide, such as GCSE, A Level, SAT, ACT, Abitur, and more. We offer an extensive library of learning materials, including interactive flashcards, comprehensive textbook solutions, and detailed explanations. The cutting-edge technology and tools we provide help students create their own learning materials. StudySmarter’s content is not only expert-verified but also regularly updated to ensure accuracy and relevance.
Learn more